CHEMISTRY AND CHEMIST1 CHEMISTRY AND CHEMIST
Without chemistry our lives would be unrecognisable, for chemistry is at work all around us. Think what life would be like without chemistry - there would be no plastics, no electricity and no protective paints for our homes. There would be no synthetic fibres to clothe us and no fertilisers to help us produce enough food. We wouldn’t be able to travel because there would be no metal, rubber or fuel for cars, ships and aeroplane. Our lives would be changed considerably without telephones, radio, television or computers, all of which depend on chemistry for the manufacture of their parts. Life expectancy would be much lower, too, as there would be no drugs to fight disease.
Chemistry is at the forefront of scientific adventure, and you could make your own contribution to the rapidly expanding technology we are enjoying. Take some of the recent academic research: computer graphics allow us to predict whether small molecules will fit into or react with larger ones - this could lead to a whole new generation of drugs to control disease; chemists are also studying the use of chemicals to trap the sun’s energy and to purify sea water; they are also investigating the possibility of using new ceramic materials to replace metals which can corrode.
Biotechnology is helping us to develop new sources of food and new ways of producing fuel, as well as producing new remedies for the sick. As the computer helps us to predict and interpret results from the test tube, the speed, accuracy and quality of results is rapidly increasing - all to the benefit of product development.
It is the job of chemists to provide us with new materials to take us into the next century, and by pursuing the subject, you could make your positive contribution to society.
Here are some good reasons for choosing chemistry as a career.
Firstly, if you have an interest in the chemical sciences, you can probably imagine taking some responsibility for the development of new technology. New ideas and materials are constantly being used in technology to improve the society in which we live. You could work in a field where research and innovation are of primary importance to standards of living, so you could see the practical results of your work in every day use.
Secondly, chemistry offers many career opportunities, whether working in a public service such as a water treatment plant, or high level research and development in industry. Your chemistry-based skills and experience can be used, not only in many different areas within the chemical industry, but also as the basis for a more general career in business.1 As a qualification, chemistry is highly regarded as a sound basis for employment.
You should remember that, as the society we live in becomes more technically advanced, the need for suitably qualified chemists will also increase. Although chemistry stands as a subject in its own right, it acts as the bond between physics and biology. Thus, by entering the world of chemistry you will be equipping yourself to play a leading role in the complex world of tomorrow.
Chemistry gives you an excellent training for many jobs, both scientific and non-scientific. To be successful in the subject you need to be able to think logically, and be creative, numerate, and analytical. These skills are much sought after in many walks of life, and would enable you to pursue a career in, say, computing and finance, as well as careers which use your chemistry directly.
Here is a brief outline of some of the fields chemists work in:
Many are employed in the wealth-creating manufacturing industries - not just oil, chemical and mining companies, but also in ceramics, electronics and fibres. Many others are in consumer based industries such as food, paper and brewing; or in service industries such as transport, health and water treatment.
In manufacturing and service industries, chemists work in Research and Development to improve and develop new products, or in Quality Control, where they make sure that the public receives products of a consistently high standard.
Chemists in the public sector deal with matters of public concern such as food preservation, pollution control, defence, and nuclear energy. The National Health Service also needs chemists, as do the teaching profession and the Government’s research and advisory establishments.
Nowadays, chemists are also found in such diverse areas as finance, law and politics, retailing, computing and purchasing. Chemists make good managers, and they can put their specialist knowledge to work as consultants or technical authors. Agricultural scientist, conservationist, doctor, geologist, meteorologist, pharmacist, vet ... the list of jobs where a qualification in chemistry is considered essential is endless. So even if you are unsure about what career you want to follow eventually, you can still study chemistry and know that you’re keeping your options open.
What Do Chemistry Graduates Do?
Demand for chemists is high, and over the last decade opportunities for chemistry graduates have been increasing. This is a trend that is likely to continue. Chemistry graduates are increasingly sought after to work in pharmaceutical, oil, chemical, engineering, textile and metal companies, but the range of opportunities also spans the food industry, nuclear fuels, glass and ceramics, optical and photographic industries, hospitals and the automotive industry. Many graduates begin in scientific research, development and design, but over the years, about half change, into fields such as sales, quality control, management, or consultancy. Within the commercial world it is recognised that, because of the general training implicit in a chemistry course, chemistry graduates are particularly adaptable and analytical - making them attractive to a very broad spectrum of employers. There has been a growth of opportunity for good chemistry graduates to move into the financial world, particularly in accountancy, retail stores, and computer software houses.
(Summarized from: A brief of the Royal Society of Chemistry,1992)
2 NOMENCLATURE OF INORGANIC COMPOUNDS
Naming elements
The term element refers to a pure substance with atoms all of a single kind. At present 107 chemical elements are known. For most elements the symbol is simply the abbreviated form of the English name consisting of one or two letters, for example:
oxygen = O nitrogen = N magnesium = Mg
Some elements, which have been known for a long time, have symbols based on their Latin names, for example:
iron = Fe (ferrum) copper = Cu (cuprum) lead = Pb (Plumbum)
A few elements have symbols based on the Latin name of one of their compounds, the elements themselves having been discovered only in relatively recent times1, for example:
sodium = Na (natrium = sodium carbonate)
potassium = K (kalium = potassium carbonate)
A listing of some common elements may be found in Table 1.
Table 1 Names of Some Common Elements
Symbol Name Symbol Name Symbol Name
Ag Silver Co Cobalt Ni Nickel
Al Aluminium Cr Chromium O Oxygen
As Arsenic Cu Copper P Phosphorus
Au gold F Fluorine Pb Lead
B boron Fe Iron Pd Palladium
Ba Barium H Hydrogen Pt Platinum
Bi Bismuth Hg Mercury S Sulfur
Br Bromine I Iiodine Se Selenium
C Carbon K Potassium Si Silicon
Ca Calcium Mg Magnesium Sn Tin
Cd Cadmium Mn Manganese Ti Titanium
Ce Cerium N Nitrogen U Uranium
Cl Chlorine Na Sodium Zn Zinc
Naming Metal Oxides, Bases and Salts
A compound is a combination of positive and negative ions in the proper ratio to give a balanced charge and the name of the compound follows from names of the ions, for example, NaCl, is sodium chloride; Al(OH)3 is aluminium hydroxide; FeBr2 is iron (II) bromide or ferrous bromide; Ca(OAc)2 is calcium acetate; Cr2(SO4)3 is chromium (III) sulphate or chromic sulphate, and so on. Table 3 gives some examples of the naming of metal compounds. The name of the negative ion will need to be obtained from Table 2.
Table 2 Some Common Negative Ions
Name Symbol Name Symbol
Nitrate NO3- Nitrite NO2-
Carbonate CO32- Sulphite SO32-
Sulphate SO42- Phosphite PO33-
Phosphate PO43- Arsenite AsO33-
Hydrogen sulphate HSO4- Hydrogen sulphite HSO3-
Hydrogen carbonate HCO3- Hypo-chlorite ClO-
Arsenate AsO43- Cyanide CN-
Iodate IO3- Iodide I-
Chlorate ClO3- Fluoride F-
Chromate CrO4- Chloride Cl-
Dichromate Cr2O72- Bromide Br-
Perchlorate ClO4- Sulphide S2-
Permanganate MnO4- Oxide O2-
Acetate OAc- Hydride H-
Oxalate C2O42- Hydroxide OH-
Table 3 Names of Some Metal Oxides, Bases and Salts
Formula Name
FeO iron(II) oxide ferrous oxide
Fe2O3 iron(III) oxide ferric oxide
Sn(OH)2 tin(II) hydroxide Stannous hydroxide
Sn(OH)4 tin(IV) hydroxide stannic hydroxide
Hg2SO4 mercury(I) sulphate Mercurous sulphate
HgSO4 mercury(II) sulphate Mercuric sulphate
NaClO sodium hypochlorite
K2Cr2O7 Potassium dichromate
Cu3(AsO4)2 copper(II) arsenate cupric arsenate
Cr(OAc)3 Chromium(III) acetate Chromic acetate
Negative ions, anions, may be monatomic or polyatomic. All monatomic anions have names ending with -ide. Two polyatomic anions which also have names ending with -ide are the hydroxide ion, OH-, and the cyanide ion, CN-.
Many polyatomic anions contain oxygen in addition to another element. The number of oxygen atoms in such oxyanions is denoted by the use of the suffixes -ite and -ate, meaning fewer and more oxygen atoms, respectively. In cases where it is necessary to denote more than two oxyanions of the same element, the prefixes hypo- and per-, meaning still fewer and still more oxygen atoms, respectively, may be used, for example,
hypochlorite ClO- Chlorite ClO2-
chlorate ClO3- perchlorate ClO4-
Naming Nonmetal Oxides
The older system of naming and one still widely used employs Greek prefixes for both the number of oxygen atoms and that of the other element in the compound 2. The prefixes used are (1) mono-, sometimes reduced to mon-, (2) di-, (3) tri-, (4) tetra-, (5) penta-, (6) hexa-, (7) hepta-, (8) octa-, (9) nona- and (10) deca-. Generally the letter a is omitted from the prefix (from tetra on ) when naming a nonmetal oxide and often mono- is omitted from the name altogether.
The Stock system is also used with nonmetal oxides. Here the Roman numeral refers to the oxidation state of the element other than oxygen.
In either system, the element other than oxygen is named first, the full name being used, followed by oxide 3. Table 4 shows some examples.
Table 4 Names of Some Nonmetal Oxides
Formula Name Name
CO carbon(II) oxide Carbon monoxide
CO2 carbon(IV) oxide Carbon dioxide
SO3 sulphur(VI) oxide Sulphur trioxide
N2O3 nitrogen(III) oxide Dinitrogen trioxide
P2O5 Phosphorus(V) oxide Diphosphorus pentoxide
Cl2O7 chlorine(VII) oxide Dichlorine heptoxide
Naming Acids
Acid names may be obtained directly from a knowledge of Table 2 by changing the name of the acid ion (the negative ion ) in the Table 2 as follows:
The Ion in Table 2 Corresponding Acid
-ate -ic
-ite -ous
-ide -ic
Examples are:
Acid Ion Acid
acetate acetic acid
perchlorate perchloric acid
bromide hydrobromic acid
cyanide hydrocyanic acid
There are a few cases where the name of the acid is changed slightly from that of the acid radical; for example, H2SO4 is sulphuric acid rather than sulphic acid. Similarly, H3PO4 is phosphoric acid rather than phosphic acid.
Naming Acid and Basic Salt and Mixed Salts
A salt containing acidic hydrogen is termed an acid salt. A way of naming these salts is to call Na2HPO4 disodium hydrogen phosphate and NaH2PO4 sodium dihydrogen phosphate. Historically, the prefix bi- has been used in naming some acid salts; in industry, for example, NaHCO3 is called sodium bicarbonate and Ca(HSO3)2 calcium bisulphite. Bi(OH)2NO3, a basic salt, would be called bismuth dihydroxynitrate. NaKSO4, a mixed salt, would be called sodium potassium sulphate.
3 NOMENCLATURE OF ORGANIC COMPOUNDS
A complete discussion of definitive rules of organic nomenclature would require more space than can be allotted in this text. We will survey some of the more common nomenclature rules, both IUPAC and trivial.
Alkanes
The names for the first twenty continuous-chain alkanes are listed in Table 1.
Table 1 Names of continuous-chain alkanes
CH4 Methane C11H24 undecane
C2H6 Ethane C12H26 dodecane
C3H8 Propane C13H28 tridecane
C4H10 Butane C14H30 tetradecane
C5H12 Pentane C15H32 pentadecane
C6H14 Hexane C16H34 hexadecane
C7H16 Heptane C17H36 Heptadecane
C8H18 Octane C18H38 Octadecane
C9H20 Nonane C19H40 Nonadecane
C10H22 Decane C20H42 Eicosane
Alkenes and Alkynes
Unbranched hydrocarbons having one double bond are named in the IUPAC system by replacing the ending -ane of the alkane name with -ene. If there are two or more double bonds, the ending is -adiene, -atriene, etc.
Unbranched hydrocarbons having one triple bond are named by replacing the ending -ane of the alkane name with -yne. If there are two or more triple bonds, the ending is -adiyne, -atriyne etc. Table 2 shows names for some alkyl groups, alkanes, alkenes and alkynes.
Table 2 Some Alkanes, Alkyl, Alkenes, Alkynes
Alkanes Alkyls Alkenes alkynes
IUPAC Common
Mthane Methyl
Ethane Ethyl Ethene Ethylene Acetylene
Propane Propyl Propene Propylene Propyne
Butane Butyl Butene Butylene Butyne
Pentane Pentyl
(amyl) Pentene Pentylene Pentyne
Hexane Hexyl
Heptane Heptyl
Octane Octyl
Nonane Nonyl
Decane Decyl
-ane -yl -ene -ene -yne
The Prefixes
In the IUPAC system, alkyl and aryl substituents and many functional groups are named as prefixes on the parent (for example, iodomethane). Some common functional groups named as prefixes are listed in Table 3.
Table 3 Some Functional Groups Named as Prefixes
Structure Name Structure Name
-OR Alkoxy- * -F Fluoro-
-NH2 Amino- -H Hydro-
-N=N- Azo- -I Iodo-
-Br Bromo- -NO2 Nitro-
-Cl Chloro- -NO Nitroso-
* methoxy-, ethoxy-, etc., depending upon the R group
In simple compounds, the prefixes di-, tri-, tetra-, penta-, hexa-, etc. are used to indicate the number of times a substituent is found in the structure: e.g., dimethylamine for (CH3)2NH or dichloromethane for CH2Cl2.
In complex structures, the prefixes bis-, tris-, and tetrakis- are used: bis- means two of a kind; tris-, three of a kind; and tetrakis-, four of a kind. [(CH3)2N]2 is bis(dimethylamino) and not di(dimethylamino).
Nomenclature Priority of Functional Groups
In naming a compound, the longest chain containing principal functional group is considered the parent. The parent is numbered from the principal functional group to the other end, the direction being chosen to give the lowest numbers to the substituents. The entire name of the structure is then composed of (1) the numbers of the positions of the substituts (and of the principal functional group, if necessary); (2) the names of the substituts; (3) the name of the parent.
The various functional groups are ranked in priority as to which receives the suffix name and the lowest position number1. A list of these priorities is given in Table 4.
Table 4 Nomenclature Priority*
Structure Name Structure Name
-N(CH3)3+ (as one example) Onium ion ArOH Phenol
-CO2H Carboxylic acid -SH Thiol
-SO3H Sulfonic acid -NR2 Amine
-COX Acid halide -O-O- Peroxide
-CONR2 Amide -MgX (as one example) Organometallic
-CN Nitrile > C=C< alkene
-CHO Aldehyde -CC- alkyne
-CO- Ketone R-, X-, etc. other substituents
ROH Alcohol
* Highest priority is at top.
Ketones
In the systematic names for ketones, the -e of the parent alkane name is dropped and -one is added. A prefix number is used if necessary.
In a complex structure, a ketone group my be named in IUPAC system with the prefix oxo-. (The prefix keto- is also sometimes encountered.)
Alcohols
The names of alcohols may be: (1) IUPAC; (2) trivial; or, occasionally, (3) conjunctive. IUPAC names are taken from the name of the alkane with the final -e changed to -ol. In the case of polyols, the prefix di-, tri- etc. is placed just before -ol, with the position numbers placed at the start of the name, if possible, such as, 1,4-cyclohexandiol. Names for some alkyl halides, ketones and alcohols are listed in Table 5.
Table 5 Some Alkyl halides, Ketones and Alcohols
Alkyl halides Ketones Alcohols
IUPAC COMMON
Chloromethane Methyl chloride Methanol
Bromoethane Ethyl bromide Ethanol
Fluropropane Propyl fluoride Acetone Propanol
Iodobutane Butyl iodide Butanone Butanol
Pentanone Pentanol
-one -ol
Ethers
Ethers are usually named by using the names of attached alkyl or aryl groups followed by the word ether. (These are trivial names.) For example, diethyl ether.
In more complex ethers, an alkoxy- prefix may be used. This is the IUPAC preference, such as 3-methoxyhexane. Sometimes the prefix- oxa- is used.
Amines
Amines are named in two principal ways: with -amine as the ending and with amino- as a prefix. Names for some ethers and amines can be found in Table 6.
Table 6 Names for Some Ethers and Amines
Ethers Amines
COMMON IUPAC COMMON IUPAC
Dimethyl ether 1-methoxy-propane Methylamine Aminomethane
Methyl ethyl ether 2-ethoxy-pentane Diethyl amine 2-amino-butane
Ethoxy-cyclo-Hexane Ethyl-methyl amine Dimethyl aminoethane
Carboxylic Acids
There are four principal types of names for carboxylic acids: (1) IUPAC; (2)trivial; (3)carboxylic acid; and (4)conjunctive. Trivial names are commonly used.
Aldehydes
Aldehydes may be named by the IUPAC system or by trivial aldehyde names. In the IUPAC system, the -oic acid ending of the corresponding carboxylic acid is changed to -al, such as hexanal. In trivial names, the -ic or -oic ending is changed to -aldehyde, such as benzaldehyde. Table 7 gives a list of commonly encountered names for carboxylic acids and aldehydes.
Table 7 Names of Some Carboxylic Acids and Aldehydes
Carboxylic acids Acids Aldehydes
Common IUPAC Common IUPAC
Formic acid Methanoic acid Formaldehyde Formyl-
(-CHO)
Acetic acid Ethanoic acid Acetaldehyde
Propionic acid Propanoic acid Propionaldehyde Propanal
Butyric acid Butanoic acid Butyraldehyde
Valeric acid Pentanoic acid
Caproic acid Hexanoic acid hexanal
Oenanthic acid Heptanoic acid
Caprylic acid Octanoic acid octanal
Pelargonic acid Nonanoic acid
Capric acid Decanoic acid decanal
Esters and Salts of Carboxylic Acids
Esters and salts of carboxylic acids are named as two words in both systematic and trivial names. The first word of the name is the name of the substituent on the oxygen. The second word of the name is derived from the name of the parent carboxylic acid with the ending changed from -ic acid to -ate.
Amides
In both the IUPAC and trivial systems, an amide is named by dropping the -ic or -oic ending of the corresponding acid name and adding -amide, such as hexanamide (IUPAC) and acetamide (trivial).
Acid Anhydrides
Acid anhydrides are named from the names of the component acid or acids with the word acid dropped and the word anhydride added, such as benzoic anhydride.
The names for some esters, amides and anhydrides are shown in Table 8.
Table 8 Some Esters, Amides and Anhydrides
Esters Amides Anhydrides
Common IUPAC
Methyl formate Formamide
Ethyl acetate Acetic anhydride
Propionamide Propanamide
Propyl butyrate N-methyl butyramide Butanamide
Acetic formic anhydride
Acid Halides
Acid halides are named by changing the ending of the carboxylic acid name from -ic acid to -yl plus the name of the halide, such as acetyl chloride.
Some names of aryl compounds and aryls are as follows:
benzene
phenyl
benzyl
aryl
benzoic acid
4. Introduction to Chemistry Department of Florida University
Program of Study
The Department of Chemistry offers programs of study leading to the M.S. and Ph.D. degrees. Students may elect studies in analytical, inorganic, organic, and physical chemistry. Specialty disciplines, such as chemical physics and quantum, bioorganic, polymer, radiation, and nuclear chemistry, are available within the four major areas.
The M.S. and Ph.D. degree requirements include a course of study, attendance at and presentation of a series of seminars, and completion and defense of a research topic worthy of publication1. Candidates for the Ph.D. degree must also demonstrate a reading ability of at least one foreign language and show satisfactory performance on a qualifying examination. The M.S. degree is not a prerequisite for the Ph.D. degree. A nonthesis degree program leading to the M.S.T. degree is offered for teachers.
Students are encouraged to begin their research shortly after selecting a research director, who is the chairman of the supervisory committee that guides the student through a graduate career.
Research Facilities
The chemistry department occupies 111,000 square feet of space in four buildings: Leigh Hall, the Chemical Research Building, Bryant Hall, and the Nuclear Science Building. Plans for a 65,000-square-foot addition to Leigh Hall are being prepared. A new central science library is located near the chemistry facilities. The University library system holds more than 2.2 million volumes.
The major instrumentation includes ultraviolet-visible, infrared, fluorescence, Roman, nuclear magnetic resonance, electron spin resonance, X-ray, ESCA, and mass spectrometers. Many are equipped with temperature-control and Fourier-transform attachments, and some have laser sources. Data-storage and data-acquiring minicomputers are interfaced to some of the instruments, such as the recently constructed quadrupole resonance mass spectrometer. The chemistry department has VAX-11/780 and VAX-11/750 computers as well as multiple terminals connected to IBM machines in the main computer centre on campus.
The departmental technical services include two well-equipped stockrooms and glassblowing, electronics, and machine shops to assist in equipment design, fabrication, and maintenance.
Financial Aid
Most graduate students are given financial support in the form of teaching and research assistantships. Stipends range from $9400 - 11,000 for the 1986-87 calendar year. State residents and assistantship holders pay in-state fees of about $1400 per calendar year. A limited number of full or supplemental fellowships are available for superior candidates.
Cost of Study
In 1985-86, in-state students paid a registration fee of $48.62, per credit hour for each semester, out-of-state students paid an additional $ 94.50 ($ 143.12 per credit hour each semester). A small increase in fees is expected for 1986-87.
5 ENVIRONMENTAL POLLUTION
With the coming of the Industrial Revolution the environmental pollution increased alarmingly. Pollution can be defined as an undesirable change in the physical, chemical, or biological characteristics of the air, water, or land that can harmfully affect health, survival, or activities of humans or other living organisms. There are four major forms of pollution - waste on land, water pollution (both the sea and inland waters), pollution of the atmosphere and pollution by noise.
Land can be polluted by many materials. There are two major types of pollutants: degradable and nondegradable. Examples of degradable pollutants are DDT and radioactive materials. DDT can decompose slowly but eventually are either broken down completely or reduced to harmless levels. For example, it typically takes about 4 years for DDT in soil to be decomposed to 25 percent of the original level applied. Some radioactive materials that give off harmful radiation, such as iodine-131, decay to harmless pollutants. Others, such as plutonium-239 produced by nuclear power plants, remains at harmful levels for thousands to hundreds of thousands of years.
Nondegradable pollutants are not broken down by natural processes. Examples of nondegradable pollutants are mercury, lead and some of their compounds and some plastics. Nondegradable pollutants must be either prevented from entering the air, water, and soil or kept below harmful levels by removal from the environment.
Water pollution is found in many forms. It is contamination of water with city sewage and factory wastes; the runoff of fertiliser and manure from farms and feed lots; sudsy streams; sediment washed from the land as a result of storms, farming, construction and mining; radioactive discharge from nuclear power plants; heated water from power and industrial plants; plastic globules floating in the world’s oceans; and female sex hormones entering water supplies through the urine of women taking birth control pills.
Even though scientists have developed highly sensitive measuring instruments, determining water quality is very difficult. There are a large number of interacting chemicals in water, many of them only in trace amounts. About 30,000 chemicals are now in commercial production, and each year about 1,000 new chemicals are added. Sooner or later most chemicals end up in rivers, lakes, and oceans. In addition, different organisms have different ranges of tolerance and threshold levels for various pollutants. To complicate matters even further, while some pollutants are either diluted to harmless levels in water or broken down to harmless forms by decomposers and natural processes, others (such as DDT, some radioactive materials, and some mercury compounds) are biologically concentrated in various organisms1.
Air pollution is normally defined as air that contains one or more chemicals in high enough concentrations to harm humans, other animals, vegetation, or materials. There are two major types of air pollutants. A primary air pollutant is a chemical added directly to the air that occurs in a harmful concentration. It can be a natural air component, such as carbon dioxide, that rises above its normal concentration, or something not usually found in the air, such as a lead compound. A secondary air pollutant is a harmful chemical formed in the atmosphere through a chemical reaction among air components.
We normally associate air pollution with smokestacks and cars, but volcanoes, forest fires, dust storms, marshes, oceans, and plants also add to the air chemicals we consider pollutants. Since these natural inputs are usually widely dispersed throughout the world, they normally don’t build up to harmful levels. And when they do, as in the case of volcanic eruptions, they are usually taken care of by natural weather and chemical cycles2.
As more people live closer together, and as they use machines to produce leisure, they find that their leisure, and even their working hours, become spoilt by a byproduct of their machines – namely, noise,
The technical difficulties to control noise often arise from the subjective-objective nature of the problem. You can define the excessive speed of a motor-car in terms of a pointer reading on a speedometer. But can you define excessive noise in the same way? You find that with any existing simple “noise-meter”, vehicles which are judged to be equally noisy may show considerable difference on the meter.
Though the ideal cure for noise is to stop it at its source, this may in many cases be impossible. The next remedy is to absorb it on its way to the ear. It is true that the overwhelming majority of noise problems are best resolved by effecting a reduction in the sound pressure level at the receiver. Soft taped music in restaurants tends to mask the clatter of crockery and the conversation at the next table. Fan noise has been used in telephone booths to mask speech interference from adjacent booths. Usually, the problem is how to reduce the sound pressure level, either at source or on the transmission path.
6 ANALYTICAL INSTRUMENT MARKET
The market for analytical instruments is showing a strength only dreamed about as little as five years ago. Driven by the need for greater chemical analysis coming from quality control and government regulation, a robust export market, and new and increasingly sophisticated techniques, sales are increasing rapidly1.
The analytical instrument business' worldwides sales are nearly double their value of five years ago, reaching $ 4.1 billion in 1987. Such growth is in stark contrast to the doldrums of several years ago when economic recession held back sales growth to little or nothing. In recent years, the instrumentation market has recovered, growing at nearly 9% per year, and it’s expected to continue at this rate at least until the 1990. With sales increases exceeding inflation, the industry has seen the real growth demonstrating the important role of chemical instrumentation in areas such as research and development, manufacturing, defense, and the environment in a technologically advancing world2.
Chromatography is the fastest-growing area, comprising 40%, or $ 1.5 billion, in 1987 world sales. Chromatographic methods are used extensively in industrial labs, which purchase about 70% of the devices made, for separation, purification, and analysis. One of the biggest words in all forms of chromatography is “biocompatibility.” Biocompatible instruments are designed to have chemically inert, corrosion-resistant surfaces in contact with the biological samples.
Gas Chromatography sales are growing at about the same rate as the instrument market. Some of the newest innovations in GC technology are the production of more instruments with high-efficiency, high-resolution capillaries and supercritical fluid capability.
Despite having only a 3% share of the GC market, supercritical fluid chromatography (SFC) has attracted a great deal of attention since its introduction around 1985 and production of the first commercial instrument around 1986. SFC, which operates using a supercritical fluid as the mobile phase, bridges the gap between GC and HPLC. The use of these mobile phases allows for higher diffusion rates and lower viscosities than liquids, and a greater solvating power than gases.
Another area showing tremendous growth is ion chromatography (IC). From growth levels of 30% per year in the U.S. and similar levels worldwide, the rate is expected to drop slightly but remain high at 25%. The popularity of IC has been enhanced through extending its applicability from inorganic systems to amino acids and other biological systems by the introduction of biocompatible instruments.
Mass spectrometry (MS) sales have been growing about 12% annually. Sales have always been high, especially since MS is the principal detector in a number of hyphenated techniques such as GC-MS, MS-MS, LC-MS, and GC-MS accounts for about 60% of MS sales since it is used widely in drug and environmental testing. Innovations in interface technology such as inductively coupled plasma/MS, SFC/MS, and thermospray or particle beam interfaces for LC-MS have both advanced the technology and expanded the interest in applications. Recent MS instruments with automated sampling and computerized data analysis have added to the attractiveness of the technique for first time users.
Spectroscopy accounts for half of all instrument sales and is the largest overall category of instruments, as the Alpert & Suftcliffe study shows. It can be broken down evenly into optical methods and electromagnetic, or nonoptical, spectroscopies. These categories include many individual high-cost items such as MS, nuclear magnetic resonance spectrometers, X-ray equipment, and electron microscopy and spectroscopy setups. Sales of spectroscopic instruments that are growing at or above the market rate include Fourier transform infrared (FTIR), Raman, plasma emission, and energy dispersive X-ray spectrometers. Others have matured and slowed down in growth, but may still hold a large share of the market.
The future of analytical instrumentation does not appear to be without its new stars as there continue to be innovations and developments in existing technology. Among these are the introduction of FT Raman, IR dichroism, IR microscopy, and NMR imaging spectrometers. Hyphenated and automated apparatus are also appearing on the market more frequently. New analytical techniques like capillary electrophoresis, gel capillary electrophoresis, scanning tunneling microscopy for the imaging of conducting systems, atomic force microscopy for the imaging of biological systems, and other techniques for surface and materials analysis are already, or may soon be, appearing as commercialized instruments. And, if the chemical industry continues to do well in the next few years, so too will the sales of analytical instrumentation.
The effect of alcohol have both medical and medicolegal implications. The estimation of alcohol in the blood or urine is relevant when the physician needs to know whether it is responsible for the condition of the patient. From the medicolegal standpoint the alcohol level is relevant in cases of sudden death, accidents while driving, and in cases when drunkenness is the defense plea. The various factors in determining the time after ingestion showing maximum concentration and the quality of the alcohol are the weight of the subject, the amount and concentration of the alcohol, how the alcohol was ingested, the presence or absence of food, and the physical state of the subject concerned1.
7 DETERMINATION OF BLOOD ALCOHOL WITH GAS CHROMATOGRAPHY
The effects of alcohol vary among individuals and for the same individuals at different times. The action depends mostly on the environment and the temperament of the individual and on the degree of dilution of the alcohol consumed. The habitual drinker usually shows relatively less effect than would be seen with an occasional drinker from the same amount of alcohol. Drugs potentiate the effect of alcohol.
Many cases document the synergistic effect of alcohol and barbiturates as a cause of death in cases appearing to be suicide. Alcohol itself is probably the most frequent cause of death due to poisoning.
A gas-solid chromatographic technique using flame ionization detection and a Porapak Q column has been used for the identification and determination of ethanol, isopropanol, and acetone in pharmaceutical preparations. The technique involves direct injection of an aqueous dilution of the product and thus is simple and direct.
Sample Preparation. Two 0.5-ml volumes of an isobutanol internal standard (10 mg/ml water; pipette 12.4 ml of isobutanol and dilute to 1 liter with water) are pipetted into two different 2-dram (7.4-ml) shell vials, one market “known.” and the other “unknown.” A 0.5-ml portion of the ethanol working standard (50 mg/100ml of blood; pipette 5ml of ethanol stock solution; dilute 12.7 ml of absolute ethanol to 1 liter with water, and dilute with 100 ml of blood from blood bank) is transferred to the vial marked “known.” The pipette is washed three times with the internal standard solution. With a 10-m l Hamilton syringe, 0.5 m l of “known” and “unknown” are injected in duplicate, and the alcohol and isobutanol peak heights are measured for each.
Gas Chromatographic Parameters. A gas chromagraph equipped with a hydrogen flame detector is used. Flame-ionization detection allows the use of samples containing water. The column is stainless steel (6ft ´ 0.25in.o.d.) packed with 30% Carbowax 20M on acid-washed 60/80-mesh Chromosorb W. The column was conditioned overnight at 180oC with a flow of nitrogen carrier gas. Operating conditions were:
Oven temperature 100 or 130oC
Injection temperature 160oC
Nitrogen flow 35ml/min
Hydrogen 28ml/min
Oxygen 100mL/min
Calculations. Peak heights of both the alcohol and the internal standard are used in the calculations. The following formula can be employed for determinations:
Where, W1 = mg ethanol
W2 = 100 ml of the unknown
Cs = Concentration of standard ethanol solution (here it is 50mg/100ml)
hx = peak height of ethanol from the unknown
hs = peak height of internal standard (isobutanol) in the known
Rx = peak height of ethanol from the unknown
Rs = peak height of internal standard (isobutanol) in the known sample
Discussion. Fig.1 shows a typical chromatogram obtained by injecting 0.5m l of blood-isobutanol internal standard mixture. The peaks are clearly separated and sharp. Table 1 gives the retention and relative retention times of some common volatile compounds associated with alcohol ingestion, diabetes, and glue sniffing.
The operating parameters such as column temperature or gas flow are not critical. They may vary with the type of compound under investigation, as well as the nature of the gas chromatograph in use.
Other packings may also be used in this determination, including Hallcomid M 180L on Chromosorb W 80/100 mesh, or Porapak Q 80/100 mesh. Porapak has different characteristics but has the advantage that it represents a packing without a coating.
Table 1 Retention Data for Some Compounds
Compound Oven Temperature 100oC Oven Temperature 130oC
Retention Time (min) Retention time Relative to EtOH Retention Time (min) Retention time Relative to EtOH
Acetaldehyde 2.56 0.73 1.25 0.60
Acetone 2.38 0.68 1.62 0.76
Chloroform 5.62 1.61 3.25 1.53
Ethanol 3.50 1.00 2.12 1.00
Isobutanol 7.25 2.10 3.94 1.86
Isopropanol 3.19 0.91 2.06 0.97
Propyl acetate 4.50 1.29 2.75 1.30
The blood that is injected into the system enters the injection port and is trapped there, while the volatiles are carried by gas flow into the column. On column injection will plug the column. Thus it would be of advantage, if this method is to be used, to have a long injection port to provide for multiple injections over a long period2. After 6-8 moths the injection port should be disconnected and the trapped blood removed, and if necessary, it is cleaned with water. The instrument should be allowed to stabilize overnight before reuse.
Quantification based on peak height is satisfactory, although peak areas may be used if the peaks obtained are too wide or are not symmetrical.
8 POLYMERS AND THEIR ADDITIVES
The term macromolecule, or polymer, is applied to substances of high molecular weight that are composed of a large number (usually at least 100) of units of low molecular weight joined by covalent bonds. If the low molecular weight units making up the macromolecule are bonded end-to-end in a long chain and no covalent chemical bonds exist between the chains, the macromolecules are called linear polymers. Such polymers, unless of extremely high molecular weight (1,000,000), can usually be dissolved and, when heated, they soften or melt so that they can be extruded into fibers or moulded into desired shapes. These polymers are said to be thermoplastic. On the other hand, if the polymer chains are linked together at numerous points, the polymer is one large three-dimensional molecule, infusible and insoluble. Such polymers are called cross-links. Certain linear polymers, referred to as thermosetting, contain groups which, when heated, react to give cross-linked polymers.1
The process by which small molecules undergo multiple combination to form macromolecules is polymerization. Small molecules from which a macromolecule or polymer can be made are called monomers. Two types of polymerization are recognized: (1)condensation polymerization and (2) addition polymerization. A polymer-forming reaction involving elimination of a small molecule such as water or alcohol between monomer units is described as condensation polymerization.2 In addition polymerization, unsaturated or cyclic molecules add to each other without elimination of any portion of the monomer molecule. The empirical formula of the polymer is then, of course, the same as that of the monomer.
Reactions capable of forming macromolecules by either addition or condensation polymerization must be functionally capable of proceeding indefinitely. Whenever two monomer molecules react, the product must contain a functional group capable of reacting with another molecule of monomer. In condensation polymerization, each monomer unit must have at least two functional groups. In addition polymerization, the monomer need have only one functional group - the presence of two or more functional groups usually leads to the production of cross-linked addition polymers.
The modulus and other physical properties of polymer can be improved by the addition of fillers and other additives, such as plasticizers, antioxidants, heat stabilizers, ultraviolet stabilizers, and flame retardants. Additives are essential functional ingredients of polymers, and whenever possible, each should be used in optimum amounts for the attainment of high-quality products.
Plasticizer: According to the ASTM-D-883 definition, a plasticizer is a material incorporated in a plastic to increase its workability and flexibility or distensibility. The addition of a plasticizer may lower the melt viscosity, elastic modulus, and glass transition temperature (Tg) of a plastic.
Antioxidants: Polymers such as polypropylene (PP) are not usable outdoors without appropriate stabilizers, because of the presence of readily removable hydrogen atoms on the tertiary carbon atoms. PP and many other polymers (RH) are attacked during processing or outdoor use in the absence of stabilizers because of a chain degradation reaction. The rate of chain-reaction degradation is retarded by the presence of small amounts of antioxidants.
Heat Stabilizers: In addition to the free-radical chain degradation described for polyolefins, another type of degradation (dehydrohalogenation) also occurs with chlorine-containing polymers such as PVC. When heated, PVC may lose hydrogen chloride and form a chromophoric conjugated polyene structure. This type of degradation can be decreased by the presence of lead, barium and cadmium salts which act as scavengers for hydrogen chloride and therefore can be used as heat stabilizers.
Ultraviolet Stabilizers: While much of the sun’s high-energy radiation is absorbed by the atmosphere, some radiation in the 280 to 400 nm (ultraviolet) range reaches the earth’s surface. Since the energy of this radiation is 100 to 72 kcal, it is sufficiently strong to cleave covalent bonds and cause yellowing and embrittlement of organic polymers. Since the effect of ultraviolet radiation on synthetic polymers is similar to its effect on the human skin, it is not surprising that ultraviolet stabilizers such as phenyl salicylate have been used for many years in suntanning lotions.
Flame Retardants: Since some polymers are used as shelter and clothing and in household furnishing, it is essential that they have good flame resistance. Combustion is a chain reaction that may be initiated and propagated by free radicals like the hydroxyl free radical. Hydroxyl radicals may be produced by the reaction of oxygen with macroalkyl radicals. Halogen radicals produced by the reaction of hydroxyl radicals with halides, such as HX, may serve as terminators for the chain reaction.
Other polymer additives are colorants, antistatic agents, foaming agents, preservatives, and processing aid or lubricants.
9 BIOCHEMISTRY AND ENZYME
Biochemistry, according to a classic definition, is the study of the chemical composition of living matter and of the chemical changes that occur in it during life processes. In perhaps a broader sense, biochemistry may be defined as a discipline in which biologic phenomena are analysed in terms of chemistry. Thus biology, including the medical and health sciences, poses the questions and, in this context, chemistry provides the intellectual and technical tools for their answer. In a very real sense, the problems that the biochemist seeks to solve are the problems of physiology, and many of the important discoveries that now fall within the scope of biochemistry were the outcome of the study of biological phenomena whose chemical aspects were only appreciated much later. Of particular importance were the accurate observations of skilled clinicians who studied the physiological dysfunctions of man.
Enzymes chemistry is one of the highlights of biochemistry study. Enzymes are a group of proteins whose biochemical function is the catalysis of chemical reactions in living systems. In living systems enzymes do not occur alone, but as part of a complex “multienzyme” apparatus. If one compares a living cell to a factory, then the individual enzymes might be considered to be analogous to the machines that cooperate to cause the transformation of a starting material (e.g., steel) into parts of a finished product (e.g., an automobile). These considerations have played an important role in the development of enzyme chemistry.
For many chemical reactions that are known to occur in living systems it is possible to assign, with reasonable certainty, single enzymes or groups of related enzymes. Consequently, it is designated a group of enzymes that have a particular type of substrate by combining the root of the substrate with the suffix -ase; thus an enzyme that acts on proteins is a proteinase, etc. Also, some enzymes are named according to the type of reaction they catalyze, such as phosphorylase or dehydrogenases. There are many instances in which a single enzyme has been referred to by means of several different names.
With few exceptions, enzyme-catalyzed reactions are performed at pH values near neutrality and at temperatures between 20 and 40oC; in order to imitate these reactions in the organic chemical laboratory, without the mediation of enzymes, more drastic conditions are usually required. In some instances such efforts may be unsuccessful, since the extreme conditions required in the absence of the appropriate enzyme may cause extensive decomposition of the components of the reaction. In general, it may be said that enzyme-catalyzed reactions are performed with a delicacy and precision that cannot be matched by the classical methods of organic chemistry.
The methods employed for enzyme isolation from cell extracts or from biological fluids are essentially those for the purification of proteins. Clearly, the same doubts must be applied to the consideration of the “purity” of crystalline enzymes as to that of crystalline proteins in general.1 With the enzymes, however, one may add to the criteria of homogeneity in solubility behaviour, sedimentation, and electrophoretic mobility, the criterion of maximal enzyme activity and freedom from other enzymatic activities. If all four of these criteria are satisfied, the probability that the enzyme is ‘pure’ is greatly increased.
In their elementary composition, the enzymes show the usual proportion of C, H, N, and S found in proteins. Some crystalline enzymes contain, in addition, small amounts of P, or metal ions such as Fe, Cu, Mg, and Zn. On hydrolysis by means of strong acids, the crystalline enzymes are largely if not completely converted to amino acids. However, although it may be said that on hydrolysis all the enzymes yield amino acids, certain of the enzymes crystallize as conjugated proteins. Thus, the "yellow enzyme" is isolated as a conjugated protein in which the non-amino acid moiety is riboflavin phosphate, while catalase is an iron-porphyrin-containing protein; the presence of the non-amino acid components is essential for the enzymatic activity of these conjugated proteins. It must be emphasized, however, that, without the intact protein, the characteristic enzymatic behaviour is absent.
10 PROCESS OF PREPARING A TEA PRODUCT (A PATENT)
19) European patent Office 11) publication number 0381462 A1
12) EUROPEAN PATENT APPLICATION
21) Application number: 84305052.7 51)Int.Cl.5 : A 23 F 3/16, A 23 F 3/20
22) Date of filing: 25.07.84
30) Priority: 29.07.83 GB 8320580 71) Application: Mars G.B.
143-149 Fenchurch Street
London EC3M 5BN(GB)
43) Date of publication of application: 72) Inventor: Smallwood, K.C.
06.03.85 Bulletin 85/10 5 Morley Road, Twickenham
Middlesex (GB)
44) Designated Contracting states: 74) Representative:
AT BE CH DE FR GB IT LI LI Colgan,Stephen James et al.,
CARMAL & RANSFORD
43 Blury Rd. London
WC1A 2RA.(GB)
54) Process of preparing a tea product.
57) In a process of preparing a tea extract, the extract is adjusted to 20-80oC , treated with tannase at pH = 4-7 and then subjected to ultrafiltration at 2-90oC using a membrane with an average molecular weight exclusion point of at least 5000 Daltons1. The extract may finally be subjected to an additional filtration, preferably microfiltration. Also an instant cold-water soluble tea powder or granulate is claimed.
The present invention relates to a process of preparing a tea extract of improved colour without turbidity. The invention also relates to a cold water-soluble instant tea powder or granulate or tea extract prepared by the process of the present invention.
The manufactured teas available on the market are usually divided into some groups, which are distinguished essentially based on the activity of tea enzymes in the course of raw tea treatment. If the enzyme activity is caused to stop at a very early stage of tea processing, then substantially unfermented or green tea is the result. A partial action of the enzymes in processing yields a yellow tea, a still greater action yields red or oolong tea and if the tea leaves are subjected to withering, rolling and sorting, almost complete fermentation, firing and final sorting, then the conventional black tea is obtained.
Black tea extracts are normally produced by a hot or boiling water extraction process, usually a countercurrent process. However, the black tea extracts, and particularly dried black tea extract, when made to beverage concentrates, usually become turbid if the beverage or the extract is allowed to cool to room temperature or lower.
This turbidity is caused by material present in the original black tea. This precipitate, known as "tea cream", is separated from the infusion, for example by centrifugation. This clouding or creaming, however, has been a serious problem in the preparation of a stable commercial tea concentrate and in the acceptance by the consumer of soluble instant tea powders, particularly of instant ice tea products.
It has been proposed, e.g. in British Patents GB-B-1,413,351 and GB-B-1,380,135 (Unilever) to remove this tea cream or to solubilise the cold water-insoluble constituents of a hot water extract of tea by treating the tea with the enzyme tannase, either in free form or fixed to an insoluble matrix.
Although the tannase treatment does give a certain amount of solubilisation of the tea cream constituents, the extract is still turbid at 5oC. The main disadvantage of this method is that the tannase comes into the tea and it has to be inactivated by a heat treatment, which is detrimental to the quality of the treated tea, or by a precipitation method which also impairs the quality of the tea.
It has now been found that the disadvantages of the prior art processes can be avoided by a method in which a tea extract, preferably a hot water tea extract, is first treated with tannase and subsequently subjected to ultrafiltration, optionally followed by a second filtration, preferably microfiltration.2
It has also been found that this method yields a product, which upon drying gives a tea extract powder with excellent cold water solubility. Normally, hot water extracts are prepared by extracting the tea with hot or boiling water (90-100oC) for a period of up to 15 min, but of course any suitable temperature-time relationship leading to a commercially satisfactory quality and yield may be used. The obtained hot tea extract is then cooled to a temperature, which make it suitable for the tannase treatment.
The enzyme tannase, which is used in the present invention, is known to hydrolyse the galloyl ester linkages of polyphenolic substrates such as tannic acid, tea polyphenol gallates, and the like. It also attacks gallic acid methyl ester. One source of the enzyme is an elaboration product of the growth of certain moulds belonging to the genus Aspergillus or Penicillium, for example Aspergillus niger, Aspergillus flavus, or Aspergillus oryzae. The method used for the determination of tannase activity of enzyme preparation is a modification of the method described by R.L. Thomas et al. in Journal of Food Science, Volume 50, 1126-1129 (1985).
The enzyme preparation can be in soluble or insoluble powder form, or in solution, or immobilised on a solid support to allow its removal from the system and reusing.
The temperature at which the treatment is carried out may, dependent on the tannase type, vary from 20 to 80oC. Very favourable yields are obtained at a temperature within the range of 40 to 70oC. The incubation time depends on the enzyme concentration and the temperature and may vary from a few minutes to several hours at lower temperatures.
An enzyme level ranging from about 0.5 to 500 or more tannase units (determined as hereinbefore described) per gram dry weight of tea may be used. In a preferred embodiment of the present invention, the tannase is recycled and in that case higher initial levels of tannase are used.
During the tannase treatment the pH drops and therefore the pH is adjusted, preferably before the conversion step to a value between 4.0 and 7.0, with a preferred pH being in the range 4.5 to 6.0.
The tea extract which has been treated with tannase is subsequently subjected to an ultra-filtration (hereafter referred to as UF) treatment. The conditions of this UF treatment are those conventionally used and are selected such that the extract is handled as carefully as possible.
The temperature at which the UF treatment is carried out is from 2 to 90oC, preferably from 5 to 40oC. The pressure in the UF treatment is generally of the order of up to 30 bar, preferably from 1 to 15 bar. The membranes used may be those conventional in the art , for example membranes of polysulphones or cellulose acetate, either in flat membrane form, tubular form, and the like. Preferably, however, tubular ceramic membranes are used. The membrane should have an average molecular weight exclusion point of at least 5,000 Daltons, preferably of at least 30,000 Daltons and most preferably of from 30,000 to 500,000 Daltons.
Although in principle a good quality of tea extract is obtained by the combination of treating tea extract with tannase followed by an UF treatment, it has been found advantageous to subject the permeate obtained in the UF treatment to an additional filtration treatment, preferably a microfiltration (hereafter referred to as MF) treatment.
To this purpose the temperature of the tea extract obtained from the UF treatment is preferably adjusted to within the range of 0 to 20° C after which the tea extract is subjected to the additional filtration treatment, preferably a MF treatment using a MF device having a mean pore size of from 0.01 to 100 micron, the pore size used being dependent on the cooling method. The obtained tea extract is then concentrated or dried in a manner known per se, for example by spray drying, or freeze drying, optionally preceded by a concentration treatment, e.g. a reverse osmosis treatment.
Claims
1. A process of preparing a tea extract, which comprises:
(a) preparing a tea extract; (b) adjusting the temperature of the extract to a value of from 20 to 80oC; (c) treating the extract with tannase while maintaining said temperature for a time sufficient to solubilise a substantial portion of the tea cream therein at a pH 4.0 to 7.0; (d) subjecting the extract to an ultrafiltration (UF) treatment at a temperature of from 2 to 90oC using a membrane having an average molecular weight exclusion point of at least 5,000 Daltons so as to obtain a retained fraction and a permeate fraction, and (e) recovering the permeate fraction.
2. A process according to Claim 1, in which the permeate fraction obtained in step (e) is subjected to the steps of: (f) adjusting the temperature of the permeate fraction to within the rang of 0-20oC; (g) subjecting the permeate fraction to a microfiltration (MF) treatment, using a MF device having a mean pore size of from 0.01 to 100 micron.
11 FOOD IRRADIATION
The process of food irradiation involves the exposure of food materials for a specified length of time to gamma-rays emitted from a radioactive source or to electrons or X-rays generated by a suitable machine. This type of radiation is known as ionising radiation because it is absorbed by the material being irradiated and generates ions which may undergo further chemical reactions with food components. In this respect ionising radiation differs from other forms of radiation such as microwaves or infrared radiation. These ions are also capable of initiating chemical reactions with constituents of micro-organisms (e.g. DNA) and it is these subsequent chemical changes that destroy cell viability and lead to the food preservative effect.
The main purpose of food irradiation is to destroy micro-organisms which cause food to become inedible or unsafe. Micro-organisms are normally found in trace amounts in foods and if not checked they may multiply rapidly. Irradiation acts by reducing the numbers of such micro-organisms.
Some micro-organisms produce substances which cause food to deteriorate. These substances are not readily destroyed by irradiation and some of them are very hazardous to health (e.g. the toxin which causes botulism). Thus irradiation cannot be used to render safe any food which has already become spoilt by the presence of toxins.
In order to ensure that food is made totally sterile high doses of irradiation would be required. This is because some micro-organisms and spores are particularly resistant to radiation. They include the most serious food poisoning micro-organism, Clostridium, botulinum. Thus, at the irradiation doses likely to be permitted most irradiated food will not be sterile and will continue to spoil. It must therefore be stored as fresh food. However it will be potentially safer and will usually keep longer.
There are a number of concerns regarding the safety of irradiation. In the first place many members of the public are ill informed about the nature of radioactivity and are unsure if food which has been irradiated may itself be made radioactive. There is also the possibility that the unusual chemical reactions that occur during irradiation may lead to the formation of chemicals that may be harmful to humans1. Concern has also been expressed about the possible loss of nutritional value of some irradiated foods. Finally it has been suggested that the small numbers of micro-organisms that do survive irradiation might in time mutate to resistant forms and so pose greater hazards to food safety.
The World Health Organization (WHO) has concluded that foods irradiated up to an overall average dose of 10 KGy (Kilogray) are nutritionally sound and safe for human consumption. When foods are irradiated at the maximum levels recommended by the WHO, vitamins B1, B6 ,C and to a less significant extent A and E, may be partially destroyed. The effects are highly variable from food to food and it is common to encounter serious disagreements between investigators considering the same foods. One obvious problem is the loss of vitamin C from potato, over and above that lost in subsequent cooking. Potato is an important source of vitamin C in the British diet although the vitamin is also obtained from other fruits and vegetables. In some special instances where the vitamin C is lost (e.g. the irradiation of fruit juices) the loss may be allowed for by fortification with the vitamin. As far as is known vitamin C is not converted to substances which may prove harmful to man but the entire range of substances to which vitamins are converted has not yet to be identified.2
It should be noted that food irradiation is not the only way in which nutrients such as vitamins B1 and C may be destroyed. For example it is well known that cooking vegetables in water leads to the loss of these vitamins as a result of the heat treatment and their solubility in the water used for cooking. The substances to which vitamins are converted as a result of irradiation or such thermal treatments are generally unknown.
The way in which irradiated food spoils is likely to be different from the way fresh food spoils because the populations of micro-organisms are modified by irradiation. Thus micro-organisms which are normally suppressed by others present in fresh foods may flourish once their competitors have been destroyed by irradiation. However the same consideration applies to the use of conventional methods of food preservation such as heat or chemical food preservatives. There is no evidence so far to suggest that micro-organisms which survive the irradiation process undergo mutations to form radiation-resistant species that are more dangerous to human health. However it is possible that some micro-organisms which normally cause food to smell or taste bad before it has become unsafe may be destroyed more effectively by irradiation than those which are hazardous to health.3 Therefore there may be no obvious sign that some irradiated foods have subsequently become unsafe to eat. This is an important aspect of food irradiation and one that requires further investigation.
The formation of new substances in food as a result of food irradiation has been the subject of research since the 1940s. There is probably more information on this matter for irradiation than for any other food preservation method. The main food component affected by irradiation is water. This is subsequently split into free radicals which then react with a wide range of food components. Several reports have mentioned that the resulting chemical products are identical to those formed during cooking. However there are reasons to question such assertions on chemical grounds and the committee felt that further information was urgently required on this matter. Generally the radicals formed disappear quickly though some may remain for periods of up to several months in material such as bone. This is because the radicals are formed and trapped in the matrix of the bone. If such bone is eaten its structure is broken down and the radicals react as previously mentioned and so disappear. It should also be appreciated that conventional cooking operations which lead to browning of foods also give rise to radicals which remain in the food and are eaten.
An example of irradiation causing an obvious chemical change in food is the inducement of rancidity in foods containing unsaturated fats when oxygen is present. The process is closely related to that which normally takes place when such foods are stored.
Some potential carcinogens and mutagens have also been identified in irradiated foods. However the levels of such substances are generally lower than in foods subjected to acceptable processes such as canning or cooking. There is no evidence from animal experiments that irradiated food is capable of causing cancer.
The type of radiation used for food irradiation is highly penetrating. Direct contamination of the food with radioactive material is not possible because the product is well packaged and held at some distance from the source. Indeed the use of an electron beam or X-rays for irradiation overcomes the need for a radioactive source altogether. Much consideration has however been given to the possibility that some atoms present in food might be converted to radioactive isotopes by the radiation. The way in which the nuclei of atoms may undergo such changes is very well understood and calculations show that any radioactivity which is induced in a worst-case situation is very small indeed. Also any such radioactivity would tend to be short lived and would quickly disappear. It is estimated that the lifetime dose of radioactivity to which a person eating irradiated food could be exposed could realistically amount to no more than one-millionth of the recognised limit of average exposure of an individual to radioactivity from other sources over the same period4. It is believed that such a level is of no significance to human health having regard to natural background levels.
The ability of radiation to induce radioactivity in food is related to its energy. This is why any regulations for food irradiation will need to specify maximum energies (e.g. 10MeV for electrons and 5MeV for X-rays). The gamma radiation sources permitted for the treatment of food, namely cobalt-60 and caesium-137 have low energies ( about 1 and 0.5MeV, respectively ). In practice it is unlikely that these energies would be exceeded in a food irradiation plant because of the lack of suitable radioactive sources or because of technical considerations associated with electron accelerators.
Summarized from: A brief of the Royal Society of Chemistry,1992
12 CHEMISORPTION OF NITROGEN ON ZSM-5 ZEOLITE (A PAPER)
Vikram S Nayak
Gulph Chemical Laboratories Ltd., 246 Silver Park Australia NIH IE7
Abstract
The present paper deals with the chemisorption of nitrogen on ZSM-5 zeolite at very high temperature. The sorption of nitrogen was measured gravimetrically using a Cahn electrobalance. The adsorption of nitrogen, which remains almost zero at temperature in the range from ambient to about 400oC, becomes appreciable at 450oC.
Introduction
The adsorption studies on ZSM-5 zeolite are generally restricted to lower temperatures. This is mainly due to the fact that most hydrocarbons upon contact with ZSM-5 zeolite undergo reaction at high temperature[1]. The study of adsorption behaviour of ZSM-5 at the catalytic reaction conditions especially when dealing with multicomponent adsorbate system is very difficult. As a step toward understanding the nature of adsorption at reaction conditions, the sorption of nitrogen at higher temperatures is studied.
Experimental Section
The adsorption of nitrogen (Matheson, 99.99%) was carried out on ZSM-5 zeolite samples which were used in earlier studies[2,3]. The sorption data were collected gravimetrically using a Cahn electromicrobalance connected to a vacuum system.
About 10-15 mg of zeolite sample was used for the sorption of nitrogen. In order to reduce the external mass-transfer resistance to the transfer of heat liberated upon adsorption, the solid sample was spread in the form of single layers of crystals over the wide surface of an aluminum sample holder. The sample could be maintained at any desired temperature with a variation of +/- 2oC by an electric furnace.
Results and Discussion
(A) Adsorption isotherm of nitrogen for ZSM-5 zeolite with Si/Al ratio 110 at 450°C
The adsorption of nitrogen on ZSM-5 zeolite above ambient temperature seems to be very much dependent upon pretreatment conditions and adsorption temperature. The adsorption of nitrogen on ZSM-5 zeolite calcined in situ at 450oC under high vacuum was almost zero at 350oC, very small at 400oC, and quite appreciable at 450oC. The adsorption isotherm of nitrogen for ZSM-5 zeolite with Si/Al ratio of 110 is shown in Figure 1A. As both adsorption (with increasing pressure) and desorption (with decreasing pressure) points lie on the common curve, the adsorption of nitrogen appears completely reversible at 450oC. In order to see the effect, if any, of Si/Al ratio on the adsorption capacity of the zeolite for nitrogen at high temperature, the adsorption experiments were carried out with ZSM-5 zeolite with Si/Al ratio of 990. The dependence of nitrogen adsorption on pressure at 450 0C for ZSM-5 zeolite with Si/Al ratio of 990 is shown in Figure 1B. The sorption isotherm and the sorption capacity of ZSM-5 zeolite with Si/Al ratio of 110 appear very much similar to those of ZSM-5 with Si/Al ratio of 990. The maximum amount of nitrogen chemisorbed at 450oC, which is about 2.75 mmol/g, corresponds to one molecule for every six framework silicon tetrahedron.
(B) Adsorption isotherm of nitrogen for ZSM-5 zeolite with Si/Al ratio 990 at 450°C
The adsorption of nitrogen at high temperature can only be explained on the basis of chemical bonding between nitrogen and the zeolite surface. It is known that the framework of ZSM-5 zeolite consists essentially of SiO2 tetrahedron with silicon atoms at the centers of the majority of the tetrahedron and aluminum atoms at the centers of the remainder small number of tetrahedron[4].
The surface of ZSM-5 zeolite is known to be covered with silanol (Si-OH) groups. Upon heating the Zeolite at high temperature (450oC) under high vacuum, a new siloxane (Si-O-Si) link is established between two silicon atoms as a result of extraction of a water molecule from the two adjacent silanol group. The new siloxane linkage can experience strain if the two silicon atoms are not sufficiently close enough for the formation of a normal siloxane linkage. Upon heating the zeolite, the normal siloxane linkage would also experience strain due to thermal expansion. The strain in the siloxane linkage, which can vary from one linkage to another, would depend mainly on the temperature at which the zeolite is held. If the strain exceeds the tolerable limit, the Si-O-Si linkage can break easily at high temperature and lead to the creation of positive and negative charges on silicon and oxygen atoms, respectively.
When the zeolite surface with strained siloxane linkages or with positive and negative charges is exposed to nitrogen at high temperature, partially dissociative adsorption of nitrogen is expected to take place.
The fact that nitrogen adsorption becomes noticeable only at high temperature is an indication that the adsorption is a highly activated process. The highly activated partially dissociative adsorption of nitrogen on ZSM-5 zeolite which is completely reversible at higher temperatures resembles to some extent the type C (M-N=N-M) nitrogen chemisorption on iron catalyst at lower temperatures.1
Acknowledgement
This work was supported in part by the National Science Foundation.
Reference
[1] Suslick K.S., New Sci., 1990, 125(1702), 50-3
[2] Bremner D., Adv.Phys.Chem., 1990, 1, 1-37
[3] Luche,J.L.,Chem.Eng., 1987, 25(1), 40-4
[4] Mason,T.J.et al., Chem.Soc.Rev., 1987, 16(2), 239-74
[5] Margulis, M.A., J.Prakt.Chem., 1990, 35(5), 579-86
Summarized from: J. Phys.Chem., 1992, 96, 8692
13 PREPARATION OF LEAD DIOXIDE ELECTRODE (A REVIEW)
W.T. Mason
(Dept. of Chem., Leeds Univ., Leeds, UK)
Introduction
Current technological developments, for instance in electro-chemical power sources, are creating fresh interest in the fundamental properties of solid oxide electrodes. Of these, lead dioxide has attracted considerable attention owing to its use as the active material in the positive plate of the lead-acid cell, and there exists a considerable amount of literature concerning the behaviour of this electrod[1-5].
For measurements on any solid electrodes the experimental requirements are severe. In the present case lead dioxide should be carefully prepared and manipulated, both mechanically and electrochemically; a rigorous standard of electrolyte cleanliness is necessary and consequently special techniques of measurement are required.
In most of the earlier reported preparations of lead dioxide no attention was paid to the polymorphic form of the product. However, in some more recent papers details of preparations are given in which careful control of the product morphology has been achieved. The various methods[6-10] for the preparation of lead dioxide that have been proposed from time to time may be subdivided into chemical and electrolytic preparations.
Lead dioxide has been prepared chemically by methods in which Pb(II) compounds are oxidized to lead dioxide in the solution phase or in melts or by heating at elevated temperatures in oxygen. It was reported that lead dioxide could be prepared by the thermal oxidation of PbO[6] or Pb3O4[7]; however, White and Roy[8] examined the products by X-ray diffraction and found that the oxide produced corresponded to an oxide with an active oxygen content of PbO[1.582]; i.e., it was not possible to produce an oxide by this method with oxygen in excess of Pb12O19. Lead dioxide may also be prepared by the hydrolysis of lead[IV] salts[8]; for example, lead tetrachloride may be hydrolyzed in cold hydrochloric acid solution[9], or a saturated solution of lead tetraacetate may be hydrolyzed in glacial acetic acid. The majority of preparation, however, involve the oxidation of lead(II) salts. Chemical oxidations of sodium plumbite solution in alkali are readily achieved with chlorine, bromine, and hydrogen peroxide[10] and simple lead(II) salts may be oxidized with 37 M nitric acid[10]. Anodic oxidations may be carried out with alkaline solutions of nitrates, perchlorates, fluoroborates, or fluorosilicates. The anodic oxidation of lead sulphate is well known[10].
Pure Lead Dioxide
The use of lead dioxide in electrolytic systems, particularly for thermodynamic measurements, has indicated that irreproducible results are often obtained, and this imposes stringent purity requirements on the materials involved.1 For example, in the work of Hamer, who studied the galvanic cell.
H2 (1 atm), Pt / H2SO4 (1M), PbSO4 , / lead dioxide, Pt
Methods for preparing lead dioxide in a suitably stable form were studied since commercial samples gave erratic emf results no matter how they were treated before use.
Hamer suggested that the electrolysis of an aqueous solution containing lead nitrate and concentrated nitric acid, maintained at 93oC with use of a platinum gauze anode, produced the most consistent potential values.
Conformation of Lead Dioxide to PbO2
A considerable amount of effort has been made into forcing lead dioxide to conform to exact stoichiometry. The methods used include chemical oxidation[12], direct oxidation at high temperatures using high oxygen pressures[13], and the crystal growth of stoichiometric lead dioxide in the solution phase[14].
Electrolytic Preparations
Of various inert materials available as anodes for the electrodeposition of lead dioxide, Pt and Au are the most suitable. Various eletrolytes have been employed. A nearly saturated solution of lead perchlorate in perchloric acid-water[14] was electrolyzed at anode current densities of 1 and 2 mA/cm2, using a platinum anode and a graphite cathode at temperatures near -50oC. Analysis of the product gave 96.4% of the theoretical active oxygen for lead dioxide, with no significant difference between materials prepared at the different current densities.
The effect of hydrogen ion concentration on the lead dioxide electrodeposit was investigated by Diusman and Giaque[10] using lead nitrate-nitric acid solutions in water. At the highest acidities, the active oxygen content of the product declined; however, there was no clear evidence that a solution of 0.1M HNO3 engendered a different product than one with 1.0 M HNO2. It was suspected that NO2- ions, formed at the cathode by reduction, may have an adverse effect on the oxygen content of the sample. This possibility was investigated and eliminated through the use of a solution of lead nitrate and copper nitrate as electrolyte, since Collat and Lingane[15] have shown that electrolytic reduction of nitrate ions proceeds all the way to NH4+ in the presence of Cu2+. No change in the active oxygen content of the samples produced was observed. Duisman and Giaque [10] also examined the effect of rotating on the anode at different speeds. It was observed that at high speeds the porosity of the sample was slightly decreased. The products, prepared at speeds higher than 100 ppm, all had essentially the same active oxygen content. Current density has no significant effect on the active oxygen content of the lead dioxide deposit. However, the samples prepared at low current densities had a more crystalline appearance and are generally of much better mechanical strength.
The existence of the two polymorphs, a -and b -lead dioxide, has been studied in great detail[14]. The following methods have been used successfully for the production of the two polymorphs.
1. a -Lead Dioxide
a) Oxidation of yellow lead monoxide by a fused sodium chlorate-sodium nitrate mixture[14]; b) Oxidation of sodium plumbite by chlorine dioxide[15]; c) Oxidation of lead acetate by ammonium persulfate[16]; d) Other methods
Other methods include alkaline formation of lead battery positive plates (Voss and Freundlich[17]) and electrooxidation of lead acetate in an alkaline solution (Zaslavskii[18]).
2. b -Lead Dioxide
b -Lead dioxide can be prepared by (i) acid formation of lead battery positive plates[13] or (ii) electrooxidation of lead perchlorate[14]. In the latter method lead monoxide (195g) was added to 500 ml of 2M perchloric acid solution and a current of density 2.5mA cm-2 was passed. The deposit was removed, ground, and washed with water.
Another preparation (iii) is electrooxidation of lead acetate in acid solution[14]. Lead acetate (100g) was dissolved in 0.5 M acetic acid solution. A platinum anode and lead cathode were suspended in the solution and a current of density 1.0 mA cm-2 was passed. The deposit was ground and washed with water.
Reference
(1) G.W.Vinal, Storage Batteries, Wiley, New York, 1965.
(2) P.Ness, Rev. Pure Applied Chem., 12, 161 (1967).
(3) C.k.Morehouse, R.Glicksman, Bull.Chem.Soc.Japan, 46,1462 (1958)
(4) J.P.Hoare, The Electrochemistry of Oxygen, Instersicience, NewYork,1969
(5) J. Burbank, A.C.Simon, Advan. Electrochem. Eng., 8, 157 (1971).
(6) R. de Levie, Can.J. Chem., 6, 329 (1967).
(7) N.V. Sidgwick, The Chemical Elements and Their Compounds, Oxford Univ. Press. London, 1950, p 118
(8) J. A. Darbyshire, J. Chem. Soc., 134, 211 (1932).
(9) W. B. White and R. Roy, J. Amer. Ceram. Soc., 47, 242 (1964)
(10) J. A. Duisman and W. F. Giaque, J. Phys. Chem., 72, 562 (1968)
(11) M. Fleischmann and H. R. Thirsk, J. Appl. Electrochem, 110, 688 (1963).
(12) W. J. Hamere, J. Amer. Chem. Soc., 57, 9 (1935).
(13) W. C. Vosburgh and D. N. Craig, ibid., 51, 2009 (1929).
(14) N. E. Bagshaw, R. L. Clarke, and B. Galiwell, J. Appl. Chem., 16, 18 (1966).
(15) N. E. Bagshqw and K. D. Wilson, Electrochim. Acta, 10, 876 (1965).
(16) N. G. Bakhchisaraits yan E. and A. Dzhafarov, Nekot., et.al., J.Colloid.Interface Sci., 10, 251,(1963)
(17) N. G. Bakchisaraits, E., A. Dzhafqrov, and G. A. Kokarev, J.Inorg.& Nucl.Chem., 32, 243 (1961).
(18) N.G. Bakchiraist; Yan E.and A. Dafrov, et.al., Microchem. J., 17, 785 (1961)
14 COORDINATION CHEMISTRY
Coordination compounds have always been a challenge to the inorganic chemist. In the early days of chemistry they seemed unusual (hence the name ¡°complex¡± ions) and seemed to defy the usual rules of valence. Today they comprise a large body of current inorganic research. A survey of articles in recent issues of the journal Inorganic Chemistry indicates that perhaps 70% could be considered to deal with coordination compounds. Although the usual bonding theories can be extended to accommodate these compounds, they still provide stimulating problems to be resolved. In synthetic work they continue to provide a challenge in the laboratory. The rapidly developing field of bioinorganic chemistry is centered on the presence of coordination compounds in living systems.
The modern study of coordination compounds begins with two men, Alfred Werner and Sophus Mads Jorgensen. Both men were astute chemists, not only in the synthetic or laboratory aspects but also in the area of interpretation and theory. As it turned out, they differed fundamentally in their interpretation of the phenomena they observed and thus served as protagonists, each spurring the other to perform further experiments to augment the evidence for his point of view. From our viewpoint a half of century later, we can conclude that Werner was ¡°right¡± and Jorgensen was ¡° wrong¡± in the interpretation of the experimental evidence they had. Indeed, Werner was the first inorganic chemist to be awarded the Nobel Prize in Chemistry (1913). Nevertheless, Jorgensen¡¯s contributions should not be slighted -- as an experimentalist he was second to none and had he not been prejudiced by some of the theories of valence current in his day, he might well have achieved the same results and fame as Werner1.
Here, an extensive discussion of the Werner-Jorgensen controversy would be out of place, but we may briefly outline the problem faced by chemists in the latter part of the nineteenth century. Many elements had fixed ¡°valences¡±, such as Na = +1, and O = -2, and some exhibited two or three stable ¡° valences¡±, e.g., Cu = +1 and +2, and P = -3, +3, and +5. Some metals, however, exhibited combining powers that were hard to reconcile with this simple picture. The standard valence of chromium was +3 and those of platinum +2 and +4. Yet chlorides of these metals will react with ammonia (in which the valences of nitrogen and hydrogen are already satisfied):
Jorgensen attempted to formulate these compounds by analogy to organic compounds, e.g.,
Werner, in formulating his ideas about the structure of coordination compounds, had before him facts such as the following2. Four ammonia complexes of cobalt (III) chloride had been discovered and named according to their colors:
One of the more interesting facts about this series is the presence of two compounds of identical empirical formula, CoCl3ž 4NH3 , but having distinct properties, the most noticeable being the difference in color. Furthermore, Werner noted that the reactivities of the chloride ions in these four compounds differed considerably. Addition of silver nitrate resulted in different amounts of precipitated silver chloride:
The correlation between the number of ammonia molecules present and the number of equivalents of silver chloride precipitated led Werner to the following conclusion:
We can thus make the general statement: Compounds M(NH3)5X3 ( M = Cr, Co; X = Cl, Br, etc. ) are derived from compounds M(NH3)6X3 by loss of one ammonia molecule. From this conclusion, Werner postulated perhaps the most important part of his theory: that in this series of compounds cobalt exhibits a constant coordination number of 6 and that as ammonia molecules are removed, they are replaced by chloride ions which now act as though they are covalently bound to the cobalt, rather than as free chloride ions3. To describe the complex chemistry of the cobalt, one must therefore consider not only the oxidation state of the metal, but also its coordination number. Werner thus formulated these four salts as [Co(NH3)6]Cl3, [Co(NH3)5Cl]Cl2 , and [Co(NH3)4Cl2]Cl.
Realizing that these formulations implied a precise statement of the number of ions formed in solution, one of Werner¡¯s first experimental studies was the measurement of the conductivities of a large number of coordination compounds.
The second important contribution that Werner made to the study of coordination chemistry was the postulate that the bonds to the ligands were fixed in space and therefore could be treated by the application of structural principles. By means of the numbers and properties of the isomers obtained, Werner was able to assign the correct geometric structures to many coordination compounds long before any direct experimental method was available for structure determination. Werner¡¯s method was that used previously by organic chemists to elucidate the structures of substituted benzenes, namely isomer counting. Werner postulated that the six ligands in an ion such as the [Co(NH3)6]+3 ion were situated in some symmetrical fashion with each NH3 group equidistant from the central cobalt atom. Three such arrangements come to mind: a planar hexagon, similar to the benzene ring, and two solid polyhedra, the trigonal prism and the octahedron. The latter is closely related to the former being formed by a 60o rotation of one of the trigonal faces. For a ¡°disubstituted¡± complex, MA4B2, the planar arrangement gives three isomers, the familiar ortho, meta, and para arrangements of organic chemistry. The trigonal prism yields three isomers also, but there are only two octahedral arrangements for this formulation.
In every case Werner investigated, the number of isomers found was equal to that expected for an octahedral complex. For [Co(NH3)4Cl2]Cl , for example, a green isomer and a violet isomer were known to Werner. Although the correlation is perfect, it must be borne in mind that the presence of two instead of three known isomers for this compound and others constitutes negative evidence concerning the structure of these complexes. Although Werner worked carefully and examined many systems, there was always the possibility, admittedly small, that the third isomer had escaped his detection. The failure to synthesize a compound, to observe a particular property, or to effect a particular reaction can never be positive proof of the nonexistence of that compound, property, or reaction. It may simply reflect some failure in technique on the part of the chemist. One well-known example of the failure of negative evidence is the overthrow of the dogma of chemical inertness on the part of the noble gases.
Werner was correct, however, in his conclusions concerning the octahedral geometry of coordination number 6 for cobalt and platinum (IV). He was also correct, and on a firmer logical footing, in his assignment of square planar geometries to the complexes of palladium and platinum from the fact that two isomers have been isolated from compounds of the formula MA2B2. The most likely alternative structure, the tetrahedron, would produce only one isomer for this composition.
Summarized from: James E Huheey, Inorganic chemistry
principles of structure and reactivity, 2nd edition, Harper & Row
15 PRODUCTION OF COKE
The process involves the production of industrial and domestic coke and related by products from the carbonisation of coal. The main process can be divided into: Coal handling, Carbonisation, Coke handling, Byproducts separation, Gas purification, Auxiliary boiler plant, Biological effluent treatment.
Coal handling: Coal is delivered by road and stored in one of three concrete floored bays. It is lifted from stock by mechanical shovel and conveyed to a 1300 tone storage bunker. From here the coal is conveyed to a 500 tone bunker located over the coke ovens.
Carbonisation: Carbonisation is the thermal process for the conversion of coal into coke, involving heating coal at high temperature for many hours in the absence of air whilst contained in ovens. A battery comprises many ovens, each oven being heated indirectly by heat transferred through its refractory walls from flues on each long wall, in which coal gas is burnt under controlled conditions in air1. Coal gas used is a proportion of that evolved from the coal during its carbonisation and after purification. Air for combustion is drawn into the battery heating system, where it is heated by passing through flues in which gas was earlier burnt, then the preheated air is introduced into individual heating flues to support combustion of gas provided through jets2. The gas burning and air preheating procedure in flues operates on a cyclic basis in which some flues are burning gas and others are giving up their heat to incoming combustion air.
Combustion gases are drawn from the oven heating flues through adjacent flues for heat recovery and are then discharged continuously to atmosphere from the battery chimney.
Prepared coal for carbonisation is introduced into each oven from its top through charging holes from a mobile charging car that runs along the length of the battery, so that it can be located above each oven in turn. On completion of charging, the coal surface is levelled in the oven by inserting a leveller bar through an oven end door and passing it along the coal surface. Immediately coal enters the oven, gases are evolved and need to be extracted from the oven to prevent emissions from the charging hole. This is achieved by extraction using a steam ejector into the gas collecting main. On completion of charging and levelling, coal is carbonised for predetermined periods of approximately 20 hours, after which hot coke is discharged from one end of the oven into a mobile coke car by means of a ram pushed through from the other end of the oven. The coke car moves quickly from the oven end along rails into a quench tower and the coke is sprayed with water.
Coke handling: Quenched coke is tipped from the coke car onto a wharf from which it is transferred by conveyor to coke cutting and size separation, from which it is put out to stock.
Byproducts separation: The gas evolved during carbonisation is collected and removed from the battery by means of an electric powered exhauster. To reduce the volume of gas handled, it has to be cooled by first spraying liquor into the collecting mains and then by passing the gases through a number of heat exchangers. The liquor is separated from the gas in a central tank, together with any condensed coal tar. The liquor is returned to the collecting main and any excess is removed for processing. Any remaining tar is removed by passing the gas through an electrostatic precipitator. All tar separated from the liquor is collected in storage tanks and transported off site for sale.
The gas then passes through two ammonia washers. In the first washer, liquor is sprayed to absorb ammonia from the gas. In the second washer, softened water is sprayed to absorb any ammonia not removed in the first washer. The resultant liquor is collected in storage tanks prior to treatment in the ammonia plant. Here ammonia is removed from the liquor and condensed to form a concentrate which is stored and dispatched off site for sale. All remaining liquor is transferred to the biological effluent treatment plant prior to discharge to sewer.
The coke oven gas, free of ammonia, is passed through two packed towers through which oil is continuously pumped. The oil removes the benzole (a mixture of benzene, toluene and xylene) from the gas stream. The benzole is then recovered from the oil by steam distillation and stored prior to dispatch off site for sale. The oil is cooled and recirculated in the scrubbing towers.
A proportion of the new debenzolised gas is returned to the battery for fuel and also to the auxiliary steam raising boilers. The remainder is either despatched off site for sale or stored in the plants gas holder. The gas holder, capacity 1400m3 allows for fluctuations in gas production. Any excess gas which cannot be sold is piped to a flare stack.
Gas purification: Crude coke oven gas contains hydrogen sulphide which is produced from sulphur in the coal. The hydrogen sulphide is removed from the gas sold by passing it through a series of boxes containing solid iron oxide. The desulphurised gas is then pumped off site via a pipeline for sale to industrial users. After a period of time, the iron oxide becomes saturated and is removed from the boxes and disposed of to landfill.
Auxiliary boiler plant: Steam for use on site is produced by burning undesulphurised coke oven gas in three boilers, producing 18,000 kg/hour steam at 7 bar gauge pressure.
Biological effluent treatment: Liquor and general process drainage is collected and treated prior to discharge to sewer. The treatment consists of mixing the effluent with biological sludge and then feeding oxygen to oxidize the polluting organic compounds to carbon dioxide. After a residence time of 24 hours, the effluent is removed from the oxidizers, the sludge is allowed to settle out and the clarified liquor is discharged to sewer.
Cooling water is used in the closed circuit system, cooled by a number of forced draught coolers. A continuous purge is currently discharged to controlled waters and made up from water pumped from the site reservoir which is fed by mines drainage or from canal3.
16 FIRST CIRCULAR OF CONFERENCE AND CALL FOR PAPERS
The 8th International Conference on Computer in Chemistry
Beijing , China, August 25-30, 1987
In chemical research and education, computers continue to play a very important role. The explosion of chemical information, the complexity of the information structure, the requirements to sophisticated instrumental facilities and the empirical nature of chemistry itself provide a wide stage of activities for computers, which are greatly enhanced by modern micro-electronics-based techniques in recent years.1 This conference is once again a gathering of the scientists in this field from all over the world to discuss advances made since the 7th ICCC in Garmisch-Partenkirchen and to discuss all the problems of common interests.
Scope
Artificial Intelligence/Expert systems, including
------Synthesis Design
------Structure Elucidation
Computer Graphics in Chemistry
Intelligent Instruments and Laboratory Automation Molecular Modelling, including:
------Quantum Chemistry and Molecular Dynamics
Personal computers, Scientific Work Stations and Super-speed Computers in Chemistry, including
------Micro-computer-based Laboratory Information Systems
------"User friendly" Software and program
------Transportation
------Optic Disk Utilization
Process Simulation, Control and Optimization in Chemical Engineering
Structure Representation and Searching for Generic Chemicals in Chemometries
Advances in Computer-aided Process Design
Plenary lectures
Professor B.Auld (Stanford University, USA)
Molecular Design of Drugs and Compounds with Desired Properties
Professor J.Kushibiki (University of Tokyo, Japan)
Data Aquisition, Evaluation, Dissemination
and Retrieval Systems
Professor A. Sarvazyan (Institute of Chemistry, Moscow, USSR)
Representation and Retrieving of Chemical Structures: Generic Chemicals and Common Data Structures
Publication
All accepted presentations (oral and poster) will be published as a book of proceeding. The 8th International Conference on Computer in Chemistry Proceeding will be published within three months after Conference and all full paying delegates will receive one copy. The abstracts of the papers will be available at the Conference.
Students Prize
A Prize of $ 50 plus a year’s subscription to Computer in Chemistry will be presented to the best student paper given at the Conference. To be considered for the award the work presented must have been undertaken by a student, and such a verification from the student’s tutor must accompany the abstract. The final decision will be made by the Organizing Panel during the Conference.
Abstracts
To take part in this important international Conference, authors must send their abstracts to the Conference Organizer by 2 November 1986. Please submit an extend and a short abstract. Extend abstract (1000 words max.): This abstract will be assessed by the Organizing Panel. It should give details of the work undertaken, pointing out novel results and relevance to the main themes of the Conference. Please provide six copies. Short abstract (300-400 words max.): The short abstract should focus on the main results of the work and will be used for the abstract book. It is important to remember that the abstract will be photographically reproduced directly from the original, so please follow these format guidelines: Type on an A4 (quarto) sheet of paper, using 12 characters per inch (or equivalent) with single spacing. Give full title, all authors (speaker underlined), full addresses and leave space between headings and text. Please provide two copies.
Exhibition
An exhibition will give interested hardware and software manufactures an opportunity to present samples of their achievements in line with the topics of the conference to international experts.
Location
The 8th International Conference on Computers in Chemistry will be held in Beijing, China’s capital and culture centre as well as a city with centuries-long history and charming scenery.
Pre-registration
For further information, particularly if you would like to receive a second circular, please return the attached reply form to the Organizing Committee:
8th ICCC Organizing Committee
Attention: Prof. Chen Qian
C/O Shanghai Institute of Organic Chemistry
345 Fengling Road
Shanghai, People’s Republic of China
The full programm of plenary and contributed papers (oral and poster) will be given in the second circular.
17 INTERNET CHEMISTRY RESOURCES
The reader can usually find a number of chemical information in encyclopedias, dictionaries, textbooks, scientific & technical data from the handbooks, tables and compendia, scientific reviews, periodicals, conference proceedings & reports, official publications, business information and abstracting and indexing periodicals from libraries. However, increasingly, chemical data are being produced in computerized form and the old manual literature searching has been significantly advanced by computer-aided searching of the literature. Online searching via the Internet and the World Wide Web has been getting a lot of attention due to very rich chemistry resources on them.
Online searching is fast. You can search thousands of documents and have answers to your questions in a matter of minutes. Online searching is convenient. You can login at your desk using a personal computer or a terminal and obtain important information whenever you need it. Online searching gives you flexibility. You can be as selective as you need to be or as inclusive as you’d like to be1. You control how much information you obtain. You can ask very simple questions. Or you can ask very complex, multifaceted questions that would be difficult or impossible to answer by consulting printed resources.
To access the chemistry resources on Internet, you need a microcomputer or terminal, a telephone line, and access to a telecommunication network through a modem with telecommunication software or a dataline to your organization’s network. You will need know some the web sites of some online search services. You will also need a login ID and password or payment for some services.
Various databases relating to the chemical literature have been developed on Internet. These databases range from storing particular information on specific compounds, to the storage of abstracts and bibliographical information of the primary and secondary literature, to actual primary sources themselves. Some examples of electronic databases on Internet that are currently used by chemical researchers are as follows.
CAS databases on STN (http://www.cas.org), produced by the American Chemical Service, is the world’s largest and most comprehensive databases of chemical information. The CAS databases, CA and REGISTRY, now include nearly 14 million abstracts of chemistry-related and patent literature and more than 17 million substances. Sources for CA include more than 8,000 journals, patents, technical reports, books, conference proceedings, and dissertations from around the world, including biochemistry, organic chemistry, macromolecular chemistry, applied chemistry and chemical engineering, physical, inorganic, and analytical chemistry.
SCI database (http://www.isinet.com), Science Citation Index, is one of the Institute for Scientific Information’s multidisciplinary cited reference databases, provide efficient access to complete bibliographic data, full author abstracts, and cited references covering over 3,300 of the world’s leading scientific and technical journals in a broad range of disciplines2. Disciplines covered agriculture, astronomy, biochemistry, biology, biotechnology, chemistry, computer science, engineering, environmental sciences, food science, genetics, geosciences, immunology, materials science, mathematics, medicine, neurosciences, oncology, pediatrics, pharmacology, physics, plant science, psychiatry, surgery, veterinary sciences, zoology.
Chemistry Information (http://www.rpi.edu/dept/chem/cheminfo/chemres.html) is an electronic reference source covering such topics as nomenclature, compound identification, proper ties, structure determination, toxicity, synthesis, registry numbers, and synthesis. It also provides definition of terms, meanings of acronyms, etc. To acquire the files, FTP to: ucssun1.sdsu.edu /pub/chemras. Use binary and the mget *.* command to transfer all files to an IBM DOS-compatible PC. Also available via WWW at URL: http://www.vuw.ac.nz/non-local/chemistry where it is called Chemical Resources.
The University of Minnesota Biocatalysis/Biodegradation Database (http://dragon.labmed.umn.edu/~lynda/index.html) is a database prototype featuring information on biocatalytic reactions and biodegradation pathways for selected chemicals. "The goal of the UM-BBD is to provide information on microbial enzyme-catalyzed reactions that are important for biotechnology." It also contains crystal data and the crystallization conditions, which have been compiled from literature. These include proteins, protein complexes, nucleic acid, nucleic acid complexes, and viruses.
Extension Toxicology Network (http://oes.orst.edu:70/1/ext/extoxnet). This source contains over 130 pesticide information profiles on specific pesticides and 18 toxicology information briefs covering selected concepts and terms in toxicology, all aimed at the nonexpert. Also answers to the questions eventually lead you to Folio, Stanford’s Campus Information System. The database contains information on hazardous properties such as flammability and corrosivity, toxic effects such as carcinogenicity, toxicity, and target organ.
JCP Express (http://jcp.uchicago.edu) is provided by the American Institute of Physics. Manuscripts submitted by authors for distribution by JCP Express have been accepted for publication in the Journal of Chemical Physics and will be available prior to publication only. They may be copied electronically and printed for individual use only. Preprints of manuscripts between acceptance and publication in the Journal of Chemical Physics. Format is PostScript and the original Text, WordPerfect, or Microsoft Word format if available.
Interactive Simulations Inc. (http://www.intsim.com/~isigen) solves critical scientific problems for the biotechnology and pharmaceutical industries, primarily through the development of innovative software for molecular modeling, simulation, and drug design.
QCPE (Quantum Chemistry Program Exchange) has long been known as a site for low-cost distribution of quantum mechanicial, molecular modeling, and other types of software. The entire holdings of QCPE, over 800 pieces of soft ware, can be searched using keywords contained in the titles of programs in the database. Search statements must be submitted as capital letters. Telnet qcpe6.chem.indiana.edu (129.79.74.206) and login as: anonymous with password: guest.
ChemViz (Chemical Visualization Project) is a NSF funded grant project to use high-powered computing and communications to help high school students better visualize abstract concepts of chemistry. Images and animations of calculated atomic and molecular orbitals can be made on Macintoshes. Contact Todd Veltman (tveltman@ncsa.uiuc.edu) for details. This also includes the periodic table database, an isotope pattern calculator, and an element percentage calculator.
The Nobel Foundation (http://www.nobel.se) provides Nobel Prize announcements and has a searchable database listing previous Nobel Laureates. The Chemical Heritage Foundation (http://beckman1.sas.upenn.edu) provides a comprehensive list of resources on the history of chemistry available through World Wide Web, as well as access to CHF's collections.
The North American Catalysis Society (http://www.dupont.com/nacs) was founded in 1956 to promote and encourage the growth and development of the science of catalysis and those scientific disciplines ancillary thereto. Society newsletters, awards and meeting information is available at this site.
Argus Chemicals, Prato, Italy (http://www.texnet.it/argus/argus.html). A producer of rare and fine chemicals, mainly organophosphorus compounds and phosphorus-based ligands. Supplier of products for electrophoretic and chromatographic separation and scale-up of proteins, peptides and nucleic acids.
Challenge, Inc. (http://www.challenge-inc.com/chem). An ISO 9002 certified chemical manufacturer specializing in process paint stripping, water-based coatings, metalworking fluids and wax emulsions.
18 SOME EXAMPLES OF CA
116: 1079770 Heat-resistant acrylic adhesives. Sugiyama, Yasuhisa; Kamio Kunimasa; Arai,Yoriyasu; Fukfda, Akio (Sumitomo Chemical Co., Ltd., Kyodo Chemical Co., Ltd.) Jpn. Kokai Tokkyo Koho JP 03,247,683 [91,247,683] (Cl.C09J163/00), 05 Nov. 1991, Appl. 90/43,935, 23 Feb. 1990; 4 pp. Oil-and heat-resistant flexible adhesives contain thermoplastic elastomers, epoxy resins, and acrylic resins contg. (A) 10-50% acrylonitrile(I) and/or methacrylonitrile, (B) 45-85% Et acrylate, Bu acrylate(II), or 2-ethylhexyl acrylate, or their mixts., and (C) 2-10% acrylic acid, methacrylic acid (III), or itaconic acid, or their mixts. Thus, I 34.0, II 57.8, and III 8.2 g were emulsion polymd. to obtain a copolymer, 30 g of which was treated with 3.6 g Sumiepoxy ESA 011(bisphenol, a epoxy resin) and 16.8 g Cariflex TR-1102 (thermoplastic SBR). The obtained viscous compn. was applied on a releasing paper to give a film,.which was hot pressed between a polyamide film and a Cu-foil to show peeling strength 1.25 kg/cm, vs. 0.30 kg/cm, for a similar compn. not contg. SBR.
116: 113525g Cyclodextrin inclusion complexes as pharmaceutical carriers. Weinsheng, Ned M. (Cyclex, Inc.) U.S. US 5,068,227 (Cl. 514-58; A61K31/70), 26 Nov 1991, Appl. 298,634, 18 Jan 1989; 23pp. Cyclodextrins(I) are coupled to biorecognition mols., such as antibodies. The cyclodextrins so coupled provide a cavity or complexation zone into which active agents such as labels or drugs may be incorporated. The active agent forms a noncovalently bonded inclusion complex within the cavity of I and thus remains assocd. with I and the coupled biorecognition mol. and thus can be delivered to the other half of the biospecific recognition.
99: 138408q Flavoring foods and chewing gums with 4,4a,5,6-tetrahydro-7-methyl-2-(3H)-naphthalenone. Pittet, Alan O.; Muralidhara, Ranya; Vock, Manfred H.; Luccarelli, Domenick, Jr.; Schmitt, Frederick L.; (International Flavors and fragrances Inc.) U.S. US 4,389,073 (Cl.426-3; A23L1/226), 24 May 1983, Appl. 362,234, 26 Mar 1982; 33 pp. Prepn. and use of 4,4a,5,6-tetrahydro-7-methyl-2-(3H)-naphthalenone (I) [34545-88-5] for enhancing the aroma or taste of foods, chewing gums, medicinal products, toothpastes, chewing tobaccos, smoking tobaccos, perfumes, colognes, detergents, fabric softeners, hair prepns, etc. are described. Thus, 3 mol of Ethyl acetoacetate [141-97-9] were treated with 2 mol of formaldehyde [50-00-0] to yield 80% of I. Addn. of I at 0.01 g to a vanilla flavor compn. enhanced the flavour by making it more natural and imparting a coumarin-like, coconut-like aroma. The formulation contg. I when added into an ice cream mix. at 0.10% gave it an excellent vanilla flavour.
116: 109364m Catalyst sintering in fixed-bed reactors: deactivation rate and thermal history. Blasco, Valero; Royo, Carlos; Monzon, Antonio; Santamaria, Jesus (Fac. Sci., Univ. Zaragoza, Zaragoza, Spain 50009). AICHE J. 1992, 38(2), 237-43 (Eng). In-situ thermal degrdn. of a com. dehydrogenation catalyst in a fixed-bed reactor is studied exptl. The activity of catalyst particles sampled at different reactor positions after several reaction-regeneration cycles is related to their time-temp. history, according to the obtained sintering kinetics under coke-free conditions.1 The results of the study show that important differences in catalytic activity can be obtained for different positions of a fixed-bed catalytic reactor after several operation-regeneration cycles.
116: 1118117e Preparation of lightweight products by a plastic method. El Shinawi, Ezzat; Nartynenko, V. V. (Egypt). Ogneupory, 1991, (9), 38-9 (Russ). A lightwt. grog thermal insulating material having apparent d. 1.0 g/cm3, compressive strength 3.0 N/mm2, and thermal cond. 0.49 and 0.55 W/m-k at 350 and 600oC resp., was prepd. from a charge contg. 50 clay and 50 vol.% sugar cane waste by firing at 1350oC for 2 h.
107: 131177p Extraction of Nicotine from tobacco waste. Nanohar, B.; Sridharan, S.S.; Univ. Coll. Technol., Osmania Univ., Hyderabad, India). CEW, Chem. Eng. World, 1987, 22(1), 89-91(Eng). Nicotine can be extd. from tobacco waste by steam distn. Process variables are studied to ext. the max. possible nicotine from tobacco dust waste contg. 1.16% nicotine. The effect of macerating time, particle size and steam velocity on the extn. yields are also studied. About 94-98% nicotine can be extd. using Ca(OH)2 as the macerating agent, and employing a steam-to-tobacco ratio of 2.5:1 to 5:1. steam distn. has to be performed immediately after the addn. of lime and water, and no effect of steam velocity on the extn. is noted, which apparently indicates that the rate of diffusion of nicotine from the tobacco particle is not a significant factor.
104: 561896p. Topical preparations containing org. semiconductors to promote peripheral vasodilation. Soma, Shihiko; Jpn. Kokai Tokkyo Koho JP 60,166,609 [85,166,609] 29 Aug 1985, 5pp. Topical prepns. (cosmetics and pharmaceuticals) contain emulsifying agents (anionic, cationic and/or nonionic surfactants) and other ingredients with addn. of org. semiconductors (Ge, Ge compds. or salts) to promote peripheral vasodilation (skin metab.) Thus, a cream was prepd. contg. liq. paraffin 10.0, lanolin 2.0, beeswax 5.0, polysorbate 80 3.5 sorbitan monofatty acid esters 1.5, water and Na4GeO4 72.0 and triethylamine 0.5%.
19 ADVERTISEMENT
(1) Job Hunting ?
BIOCHEMIST: Applications are invited for a tenure track position in the Department of Chemistry at Whitman College, a private liberal arts college offering an ACS-certified BA. A recent Ph.D. in chemistry or biochemistry is required. Principal duties include teaching an undergraduate biochemistry course, one section of general chemistry lecture and laboratory, a special topics course in biochemistry, and the direction of undergraduate chemistry research projects. Applications (including transcripts of academic work), three letters of recommendation, curriculum vitae, and a description of teaching experience and philosophy and research interests should be sent to Professor David L.Frasco, Chair, Department of Chemistry, Whitman college, Walla, WA99362, by January 15, 1989. Rank and salary dependent upon experience. Whitman is an AA/EO employer.
(2) To Order New Book ?
ANNUAL REPORTS IN ORGANIC SYNTHESIS -1982
Volume 13
Edited by L.G. WADE
Colorado State University, Fort Collins
1983, 520 pp., USD 29.00/ISBN:0-12-040813-9
CONTENTS: Carbon-carbon Bond Forming Reactions: Carbon-Carbon Single Bonds. Carbon-Carbon Double Bonds. Carbon-Carbon Triple Bonds. Thermal Reactions. Aromatic Substitutions Forming a New Carbon-Carbon Bond. Synthesis via Organometallics. Oxidations: C-O Oxidations. C-H Oxidations. C-N Oxidations. Amine Oxidations, Sulphur Oxidations. Oxidative Additions to C-C Multiple Bonds. Phenol - Quinone Oxidation. Oxidative Cleavages. Photosensitized Oxygenation. Dehydrogenation. Other Oxidations and Reviews. Reductions: C = O Reductions. Nitrile Reductions. Reduction of Sulphur Compounds. N-O Reductions. C-C Multiple Bond Reductions. Hydrogenolysis of Hetero Bonds. Reductive Eliminations. Reductive Cleavages. Other Reductions and Reviews. Synthesis of Heterocycles: Furans, etc. Indoles. Lactams. Lactones. Pyridines, Quinolines, etc. Pyrroles, etc. Other Heterocycles with one Heteroatom. Heterocycles with Two or More Heteroatoms. General Reviews. Protecting Groups: Hydroxyl. Amine. Sulf-hydryl. Carboxyl. Ketone. Aldehyde. Phosphate. Miscellaneous Protecting Groups. Useful synthetic preparations: Functional Group Preparations. Ring Enlargement and Contraction. Multistep Transformations. Miscellaneous Reviews. Author Index.
Please check box 5 on the order card.
(3) MSA-The Ideal Electrolyte for Electroplating
Methane sulphonic acid (MSA) has the right combination of properties for electroplating baths containing lead, tin, copper, nickel and some precious metals. It is non-oxidizing, provides the necessary solubility, will not chelate metal ions, and has relatively low toxicity.
Because MSA is non-complexing, it reduces the cost of complex waste treatment to bring tin and lead levels in the wastewater into compliance with environmental regulations.1 Further, the mesylate ion is considerably less toxic (LD50 = 6300mg/kg).
These advantages suggest the use of MSA in virtually any plating operation involving solder or lead. MSA is also the electrolyte of choice for wire coating with tin or for Babbitt metal plating applications.
Pennwalt is the leading producer of MSA and has recently completed a major plant expansion in Wyandotte, Michigan.
For additional information about MSA in electroplating applications, fill in the reply card or contact your nearest Pennwalt Sales office.
(4) Digital Self-tuning Temperature Controller (Model 290)
Easy to set push-button digital temperature control for heaters up to 1800W. Self-tuning algorithm automatically controls temperature with minimal overshoot and ripple. The controller has a completely independent, built-in, backup controller to guard against heating accidents from equipment failure. In the event that one controller fails, the other take over. The desired temperature is entered into the digital display of the first controller, which regulates heating. If the reaction reaches the high temperature limit entered into the second controller, power is removed from the output receptacles until the unit is manually reset. Both controllers independently monitor the reaction temperature for 100% redundant control. Displays “set” and “actual” apparatus temperature. Controller automatically turns off heater if thermocouple fails or disconnects. Nonvolatile memory retains temperature settings. Double-fused for safety. Controller includes a dual-element thermocouple (1/4 in.diam._12in.L; Teflon-coated), dual element thermocouple extension cord (10ft), and a Teflon probe adapter with T 14/20 joint. Specs: 15amps @ 120VAC; 1800W. Dim.: 3.25L´ 7.5W´ 9in.H.
20 A CONTRACT TO SELL ZINC
ORIGINAL
Contract No. 92YCYN-25
Signing Date: Sep.8, 1992 KUNMING, CHINA
The Seller: CHINA METALLURGICAL IMPORT &
EXPORT CORP. YUNNAN BRANC
Add: 14 RENMIN DONG ROAD KUNMING CHINA
Tel: 22971 21386 26679 26668
FAX: (0871)-20479
Cable: 4000 KUNMING ,
TEL: 64035 MIECY CN
The Buyer: NARA RESOURCE LTD
. RM.805, NAMKANG BLDG 32-2, JUNE-KU, SEOUL,
KOREA
Cable: Telex: K35927
The Seller agrees to sell and the Buyer agrees to buy the undermentioned goods on the terms and conditions stated below:
(1) Description of
Commodity, Sepcifications (2) Quantity (3) Unit Price (4) Total Amount
DESCRIPTION:
ZINC INGOT.
SPEC: ZN:99% MIN. -480-MT USD1,120.-/MT CNF INCHON, KOREA USD 537,600.00
SHIPMENT 10% MORE OR LESS AT SELLER'S OPTION
(5) Packing Term: IN BUNDLE WITH STEEL STRIP, ABOUT 0.8-1.0 MT NET EACH AND INTO CONTAINERS.
(6) Shipping Marks: AT SELLER’S OPTION.
(7) Port of Loading: China Ports CHINA MAIN PORT.
(8) Port of Destination: INCHON, KOREA.
(9) Time of Shipment:-180-MT WITHIN OCT.1992 -0300-MT WITHIN NOV.1992
(10) Insurance: TO BE EFFECTED BY THE BUYERS.
(11) Inspection: INSPECTION CERTIFICATE OF QUALITY AND WEIGHT ISSUED BY CCIB.
(12) Terms of Payment: L/C AT SIGHT.
The Buyer shall open, with a bank to be accepted by both the Buyer and the Seller, an irrevocable, transferable and divisible Letter of Credit at sight, allowing partial shipments and transhipment, in favour of the Seller (CHINA METALLURGICAL IMP. AND EXP. CORPORATION YUNNAN BRANCH) and negotiable against first presentation of the shipping documents to the Bank of China (YUNNAN BRANCH) in China.2 The covering Letter of Credit must be opened and reached to the Seller before _____________ and to remain valid in China until the 20 day (inclusive) from the date of shipment.
(13) Documents: The Seller shall present to the negotiating bank, Clean On Board Bill of Loading (or Clean On Board Bill of Loading for container transportation), Quality certificate, Survey Report on Quantity/Weight, and Transferable Insurance Policy or Insurance Certificate when this Contract is made on CIF basis.2
(14) Terms of Shipment (CIF/C&F):
The carrying vessel shall be provided by the Seller. Partial shipments, transhipment and container transportation are allowed.
After loading is completed, the Seller shall notify the Buyer by CABLE of the contract number, description of commodity, quantity, name of the carrying vessel and date of shipment.
(15) Quality/Quantity/Weight Discrepancy and Claim:
In case the quality and/or quantity/weight are found by the Buyer to be not in conformity with the Contracts after arrival of the goods at the port of destination, the Buyer may lodge claim with the Seller supported by survey report issued by an inspection organization agreed upon by both parties, with the exception, however, of those claims for which the insurance company and/or the shipping company are to be held responsible. Claim for quality discrepancy should be filed by the Buyer with 30 days after arrival of the goods at the port of destination, while for quantity/weight discrepancy claim should be filed by the Buyer within 15 days after arrival of the goods at the port of destination. The Seller shall, within 30 days after accept of the notification of the claim, send reply to the Buyer.
(16) Force Majeure: In case Force Majeure, the Seller shall not be held responsible for late delivery or nondelivery of the goods but shall notify the Buyer by cable. The seller shall deliver to the Buyer by registered mail, if so requested by the Buyer, a certificate issued by the China Council for the Promotion of International Trade or any competent authorities.
(17) Arbitration: All disputes in connection with this Contract or the execution thereof shall be settled through friendly negotiations between two parties. If no settlement can be reached, the case shall then be submitted to the Foreign Trade Arbitration Commission of the China Council for the Promotion of the International Trade, Beijing, for settlement by arbitration in accordance with the Commission’s Provisional Rules of Procedure. The award rendered by the Commission shall be final and binding on both Parties. The arbitration expenses shall be borne by the Losing party unless otherwise awarded by the arbitration organization.3
(18) Addition clauses: THE SELLER HAVE TO SEND FOR ANY COMMISSION IN TWO TIMES TO NARA RESOURCE LTD BY T/T WITHIN 20 DAYS AFTER SHIPMENT.
Seller Buyer
YUNNAN METALLURGICAL IMPORT AND EXPORT CORPORATION KIM CHANGON NARA RESOURCE LTD
Appendix 1 Name and Symbols of Elements 元素的英文名及符号
At.No. Name 汉语名 At.No. Name 汉语名
1 Hydrogen 氢 56 Barium 钡
2 Helium 氦 57 Lanthanum 镧
3 Lithium 锂 58 Cerium 铈
4 Beryllium 铍 59 Praseodymium 镨
5 Boron 硼 60 Neodymium 钕
6 Carbon 碳 61 Promethium 钷
7 Nitrogen 氮 62 Samarium 钐
8 Oxygen 氧 63 Europium 铕
9 Fluorin 氟 64 Gadolinium 钆
10 Neon 氖 65 Terbium 铽
11 Sodium 钠 66 Dysprosium 镝
12 Magnesium 镁 67 Holmium 钬
13 Aluminium 铝 68 Erbium 铒
14 Silicon 硅 69 Thulium 铥
15 Phosphorus 磷 70 Ytterbium 镱
16 Sulfur 硫 71 Lutetium 镥
17 Chlorine 氯 72 Hafnium 铪
18 Argon 氩 73 Tantalum 钽
19 Potassium 钾 74 Tungsten 钨
20 Calcium 钙 75 Rhenium 铼
21 Scandium 钪 76 Osmium 锇
22 Titanium 钛 77 Iridium 铱
23 Vanadium 钒 78 Platinum 铂
24 Chromium 铬 79 Gold 金
25 Manganese 锰 80 Mercury 汞
26 Iron 铁 81 Thallium 铊
27 Cobalt 钴 82 Lead 铅
28 Nickel 镍 83 Bismuth 铋
29 Copper 铜 84 Polonium 钋
30 Zinc 锌 85 Astatine 砹
31 Gallium 镓 86 Radon 氡
32 Germanium 锗 87 Francium 钫
33 Arsenic 砷 88 Radium 镭
34 Selenium 硒 89 Actinium 锕
35 Bromine 溴 90 Thorium 钍
36 Krypton 氪 91 Protactinium 镤
37 Rubidium 铷 92 Uranium 铀
38 Strontium 锶 93 Neptunium 镎
39 Yttrium 钇 94 Plutonium 钚
40 Zirconium 锆 95 Americium 镅
41 Niobium 铌 96 Curium 锔
42 Molybdenum 钼 97 Berkelium 锫
43 Technetium 碲 98 Californium 锎
44 Ruthenium 钌 99 Einsteinium 锿
45 Rhodium 铑 100 Fermium 镄
46 Palladium 钯 101 Mendelevium 钔
47 Silver 银 102 Nobelium 锘
48 Cadmium 镉 103 Lawrencium 铹
49 Indium 铟 104 Rutherfordium
50 Tin 锡 105 Dubnium
51 Antimony 锑 106 Seaborgium
52 Tellurium 锝 107 Bohrium
53 Iodine 碘 108 Hassium
54 Xenon 氙 109 Meitnerium
55 Cesium 铯
Appendix 2 Common Glassware Names
常见玻璃器皿名称
adapter 接液管
air condenser 空气冷凝管
beaker 烧杯
boiling flask 烧瓶
boiling flask-3-neck 三口烧瓶
burette clamp 滴定管夹
burette stand 滴定架台
Busher funnel 布氏漏斗
Claisen distilling head 减压蒸馏头
condenser-Allihn type 球型冷凝管
condenser-west tube 直型冷凝管
crucible tongs 坩埚钳
crucible with cover 带盖的坩埚
distilling head 蒸馏头
distilling tube 蒸馏管
Erlenmeyer flask 锥型瓶
evaporating dish (porcelain) 瓷蒸发皿
filter flask(suction flask) 抽滤瓶
florence flask 平底烧瓶
fractionating column 分馏柱
Geiser burette (stopcock) 酸氏滴定管
graduated cylinder 量筒
Hirsch funnel 赫氏漏斗
long-stem funnel 长颈漏斗
medicine dropper 滴管
Mohr burette for use with pinchcock
碱氏滴定管
Mohr measuring pipette 量液管
mortar 研钵
pestle 研杵
pinch clamp 弹簧节流夹
plastic squeeze bottle 塑料洗瓶
reducing bush 大变小转换接头
rubber pipette bulb 吸耳球
screw clamp 螺旋夹
separatory funnel 分液漏斗
stemless funnel 无颈漏斗
test tube holder 试管夹
test tube 试管
Thiele melting point tube 提勒熔点管
transfer pipette 移液管
tripod 三角架
volumetric flask 容量瓶
watch glass 表皿
wide-mouth bottle 广口瓶
Appendix 3 Common Chemical Abbreviation
化学上常见的缩略语
ab. absolute 绝对的
addn. addition 添加
alc. alcohol 醇
alk. alkali 碱
amt. amount 量
A.P. analytically pure 分析纯
app. apparatus 装置
approx. approximate 大约
aqu. aqueous 水的
asym. asymmetric 不对称的
atm. atmospheric 大气压
av. average 平均的
b.p. boiling point 沸点
ca. about 大约
cal. caloric 卡路里
calc. calculate 计算
cf. compare 比较
chem. chemistry 化学
conc. concentrated 浓缩的
const. constant 常数
contg. containing 含有…的
compd. compound 化合物
C.P. chemically pure 化学纯
cryst. crystalline 晶体
decomp. decompose 分解
deriv. derivative 衍生
detn. determination 测定
dil. dilute 稀释的
distd. distilled 蒸馏的
e.g. for example 例如
elec. electric 电的
eq. equation 方程
equil. equilibrium 平衡
equiv. equivalent 等价的
et. al. and others 以及其他人
etc. et cetera 等等
evap. evaporation 蒸发
expt. experimental 实验的
fig. figure 图
hyd. hydrous 水的
ibid. in the same place在同一地方
lab. laboratory 实验室
liq. liquid 液体
L.R. laboratory reagent实验试剂
manf. manufacture 制造
max. maximum 最大的
min. minute 最小的
mixt. mixture 混合物
mol.wt. molecular weight 分子量
m.p. melting point 熔点
org. organic 有机的
ppm. parts per million百万分之一
ppt. precipitated 沉淀的
prep. prepare 制备
resp. respectively 分别地
sec. second 第二
soln. solution 溶液
solv. solvent 溶剂
sp.gr. specific gravity 比重
sq. square 平方
sub. sublime 升华
susp. suspended 悬浮地
tech. technical 技术的
Tech.P. technically pure 技术纯
temp. temperature 温度
vol. volume 体积
wt. Weight 重量
Appendix 4 Common Chemical Prefix and Suffix
化学上常用的词头及词尾的含义
-acetal 醛缩醇
acetal- 乙酰
acid 酸
-al 醛
alcohol 醇
-aldehyde 醛
alkali- 碱
allyl 丙烯基
alkoxy- 烷氧基
-amide 酰胺
amino- 氨基的
-amidine 脒
-amine 胺
-ane 烷
anhydride 酐
anilino- 苯胺基
aquo- 含水的
-ase 酶
-ate 含氧酸的盐、酯
-atriyne 三炔
azo- 偶氮
benzene 苯
bi- 在盐类前表示酸式盐
bis- 双
-borane 硼烷
bromo- 溴
butyl 丁基
-carbinol 甲醇
carbonyl 羰基
-caboxylic acid 羧酸
centi- 10-2
chloro- 氯代
cis- 顺式
condensed 缩合的、冷凝的
cyclo- 环
deca- 十
deci 10-1
二
-dine 啶
dodeca- 十二
-ene 烯
epi- 表
epoxy- 环氧
-ester 酯
-ether 醚
ethoxy- 乙氧基
ethyl 乙基
fluoro- 氟代
-form 仿
-glycol 二醇
hemi- 半
hendeca- 十一
hepta- 七
heptadeca- 十七
hexa- 六
hexadeca- 十六
-hydrin 醇
hydro- 氢或水
hydroxyl 羟基
hypo- 低级的,次
-ic 酸的,高价金属
-ide 无氧酸的盐,酰替…胺,酐
-il 偶酰
-imine 亚胺
iodo- 碘代
iso- 异,等,同
-ite 亚酸盐
keto- 酮
ketone 酮
-lactone 内酯
mega- 106
meta- 间,偏
methoxy- 甲氧基
methyl 甲基
micro- 10-6
milli- 10-3
mono- ( mon-) 一,单
nano- 10-9
nitro- 硝基
nitroso- 亚硝基
nona- 九
nonadeca- 十九
octa- 八
octadeca- 十八
-oic 酸的
-ol 醇
-one 酮
ortho- 邻,正,原
-ous 亚酸的,低价金属
oxa- 氧杂
-oxide 氧化合物
-oxime 肟
oxo- 酮
oxy- 氧化
-oyl 酰
para- 对位,仲
penta- 五
pentadeca- 十五
per- 高,过
petro- 石油
phenol 苯酚
phenyl 苯基
pico- 10-12
poly- 聚,多
quadri- 四
quinque- 五
semi- 半
septi- 七
sesqui 一个半
sexi- 六
sulfa- 磺胺
sym- 对称
syn- 顺式,同,共
ter- 三
tetra- 四
tetradeca- 十四
tetrakis- 四个
thio- 硫代
trans- 反式,超,跨
tri- 三
trideca- 十三
tris- 三个
undeca- 十一
uni- 单,一
unsym- 不对称的,偏位
-yl 基
-ylene 撑(二价基,价在不同原子上)
-yne 炔
Appendix 5 Common Prefix and Suffix 常用词头及词尾的含义
ab- 脱离
-able 能…的
ad- 添加
anti- 反对,抗
auto- 自
bi- 二,双
by- 附属的,次要的
centi- 百,百分之一
circum- 环境, 在周边
co- 共,一齐
con- 共同,一起
contra- 反对,相反
counter- 逆,反
de- 除去
deca- 十
deci- 十分之一
di- 二
dia- 通过,横过
dis- 不,无,解除
en- 使,置于
-en 加在形容词后,表“使…”
equi- 同等
ex- 除去,离开,出自
-fold 加在数词后,表示“…倍”
-free 无…的
-ful 充满…的
-graph 名词词尾,写、画的结果或用具
hecto- 百
hemi- 半
hetero- 异,杂
hexa- 六
homo- 同
hydro- 水
hyper- 超越
hypo- 低,次
-ician 名词词尾,…精通者,…家
-ics 名词词尾,…学
-ify 动词词尾,使…,…化
in- 在内,向内,不
inter- 在…之间,相互
intro- 向内
-ish 形容词词尾,略带…色的
-ism …学说
-ist …的信仰者
-ive 形容词词尾,易…的
-ize 动词词尾,…化,变成…
-less 无…的
-logy …学
kilo- 千
mega- 大,兆,百万
-meter …计,…表
micro- 微,小,百万分之一
mid- 中
mis- 误
milli- 千分之一,毫
mono-, 单,一
multi- 多
non- 非,不
nona- 九
oct- ,octa- 八
out- 超过,向外
over- 超过,过分
pent- 五
per- 高,过
peri- 周围,近,环境
phono- 声
photo- 光
poly- 多,聚
post- 在后,补充
pre- 预先,在前
pro- 向前
-proof 防…的
quadr- 四
quinque- 五
re- 再次,重复, 反对,返回
radio- 放射,无线电
-scope …镜
semi- 半
sex- 六
sept- 七
sub- 次于,在下,低,稍微
sur- 在上,超
super- 超,过
syn- 或 sym- 共,同
tele- 远
tetra- 四
-tight 紧密的
trans- 横过,转移
tri- 三
ultra- 超,极端
un- 不,未
uni- 单,一
under- 不足,在…下
with- 反对,返
Appendix 6 Speaking of Common Molecular Formulas, Equations and Mathematical Symbols
常用化学分子式、方程式及数学式的读法
Na2SO4 Sodium sulphate; C-a-two-S-O-four
Mg(OH)2 magnesium hydroxide; M-g-pause-O-H-pause-twice; M-g-in brackets twice
Ca2(PO4)3·2H2O calcium phosphate two hydrate; C-a-two-pause-P-O four-pause- three times-dot-two-H two-O
[Zn(NH3)4]2+ tetra-ammonium zinc complex cation
→ give; yield; produce, form,become
↑evolved as a gas; give off a gas
↓is precipitated; gives X precipitate
reacts reversibly
Cu, △ in the precence of a copper as a catalyst on heating
CO32- + Ca2+ = CaCO3↓ a carbonate anion with a valancy of two plus a calcium cation with a valancy of two produce a calcium carbonate precipitate
R’ R prime
R” R double prime, R second prime
R1 R sub one
100℃ one hundred degrees Centigrade
+ plus; positive
- minus; negative
× multiplied by; times
÷ divided by
± plus or minus
= is equal to; equals
≡ is identically equal to
≈ is approximately equal to
( ) round brackets; parentheses
[ ] square brackets
{ } braces
a>>b a is much greater than b
a≥ b a is greater than or equal to b
a∝b a varies directly as b
lognX logX to the base n
the cubic root of x
the nth root of x
x2 x square, x squared, the square of x
Xn X to n factors; the nth power of x; x to the power n
x -8 x to the minus eighth power
| x| the absolute value of x
the mean value of X
● the sum of the terms indicated; summation of
△x or δx the increment of x
dx differential x
dy/dx the first derivative of y with respect to x
∫ integral
infinity
1/2 a half; one half
2/3 two thirds
5/123 five over a hundred and twenty-three
eight and three over fourths; eight and three quarters
0.01 O point O one; zero point zero one; nought point nought one
6 % 6 percent
3‰ 3 per mille
2 : 3 the ratio of two to three
r=xd r equals x multiplied by d
52=10 five times two equals ten
x3/8=y2 x raised to the third power divided by eight equals y squared
(a+b-c d)/e=f a plus b minus c multiplied by d, all divided by e equals f
y = (Wt-W)/x y equals W sub t minus W over x
Notes
1 Your chemistry-based skills and experience can be used, not only in many different areas within the chemical industry, but also as the basis for a more general career in business. 商界已公认,由于在化学课程中得到了全面的训练,化学专业毕业生们的适应性及分析能力都很强,这使得他们倍受各行各业雇主的青睐.。
2 (1) A few elements have symbols based on the Latin name of one of their compounds, the elements themselves having been discovered only in relatively recent times. 该句中, “A few elements ..... compounds” 是主句, “ the elements .... recent times”系独立主格结构,用作附加说明。 本句译文: 一些元素的符号是由他们的一种化合物的拉丁语名称命名的,而这些元素本身相对来说是近代才被发现的。
(2) The older system of naming and one still widely used employs Greek prefixes for both the number of oxygen atoms and that of the other element in the compound. 句中 one 指前面的System, that 代替前面的the number。 本句译文: 仍广泛使用的老式命名法是对化合物中的氧原子和其它元素的数量使用希腊词词头。
(3) In either system, the element other than oxygen is named first, the full name being used, followed by oxide. “ the full ... used” 系独立主格结构, “followed by oxide” 是方式状语。 本句译文: 在任何一种体系中,都是首先命名氧之外的元素, 使用该元素的全称,接着是oxide( 氧化物)。
3 The various functional groups are ranked in priority as to which receives the suffix name and the lowest position number. 各种官能团按次序优先原则排列,以便确定后缀及最小位数。
4 (1) The M.S. and Ph.D. degree requirements include a course of study, attendance at and presentation of a series of seminars, and completion and defense of a research topic worthy of publication. 获硕士及博士学位的要求包括课程学习,参加一系列专题讨论会以及完成一个有发表价值的研究课题并通过答辩。
5 (1) To complicate matters even further, while some pollutants are either diluted to harmless levels in water or broken down to harmless forms by decomposers and natural processes, others (such as DDT, some radioactive materials, and some mercury compounds) are biologically concentrated in various organisms. 使得事情更加复杂化的是,当一些污染物在水中被稀释到无害的水平或被分解体几自然过程分解为无害的形式时,另一些污染物(如滴滴梯、放射性物质和汞化合物)却被各种生物所富积。
(2) And when they do, as in the case of volcanic eruptions, they are usually taken care of by natural weather and chemical cycles. 当自然投入物积累到有害程度时(如火山喷发),它们通常也能够被自然气候和化学循环所清除。
6 (1) Driven by the need for greater chemical analysis coming from quality control and government regulation, a robust export marked, and new and increasingly sophisticated techniques, sales are increasing rapidly. 在对由于实行质量控制及政府法规所需要的化学分析的增多,出口市场的活跃以及越来越复杂的新技术等需要的驱使下,销售正迅速增长。(2) With sales increases exceeding inflation, the industry has seen real growth demonstrating the important role of chemical instrumentation in areas such as research and development, manufacturing, defense, and the environment in a technologically advancing world. 随着贸易的增长超过了通货膨胀,工业界看到了在一个技术不断进步的世界里,化学仪器在各个领域(如在研究与开发、制造业、 国防工业及环保产业)中的重要作用也正不断增长。
7 (1) The various factors in determining the time after ingestion showing maximum concentration and the quality of the alcohol are the weight of the subject, the amount and concentration of the alcohol, how the alcohol was ingested, the presence or absence of food, and the physical state of the subject. 决定摄入酒后显示出最大乙醇浓度及量的时间的因素是:对象的体重、乙醇的量及浓度、摄入乙醇的方式、有无食物存在以及对象的身体状况。
(2) On column injection will plug the column. Thus it would be of advantage, if this method is to be used, to have a long injection port to provide for multiple injections over a long period. 在柱上进样将堵塞柱子,所以,如果使用此法,选一个长的进样阀将有利于多次进样并能延长使用周期。
8 (1) Certain linear polymers, referred to as thermosetting, contain groups which, when heated, react to give cross-linked polymers. 某些被认为是热固性的线性聚合物含有一些官能团,这些官能团在加热时发生反应,产生交联聚合物。
(2) A polymer-forming reaction involving elimination of a small molecule such as water or alcohol between monomer units is described as condensation polymerization. 涉及在聚合物单体间消除一个小分子、如水或醇而形成聚合物的反应,称为缩聚反应。
9 (1) Clearly, the same doubts must be applied to the consideration of the "purity " of crystalline enzymes as to that of crystalline proteins in general. 不言而喻, 一般说来对蛋白质晶体“纯度”产生的疑问同样也适用于晶体酶的情况。
10 (1) In a process of preparing a tea extract, the extract is adjusted to 20-80oC , treated with tannase at pH = 4-7 and then subjected to ultrafiltration at 2-90oC using a membrane with an average molecular weight exclusion point of at least 5000 Daltons. 在一个制备茶提取物的工艺中,将茶提取物的温度调节在20-80℃之间, 在pH= 4-7下用单宁酶处理,然后,用平均分子量至少大于5000道尔顿的滤膜在2-90℃下进行超过滤。
(2) It has now been found that the disadvantages of the prior art processes can be avoided by a method in which a tea extract, preferably a hot water tea extract, is first treated with tannase and subsequently subjected to ultrafiltration, optionally followed by a second filtration, preferably microfiltration. 现已发现,先前工艺的缺点可用下述方法加以避免,即将茶的萃取物首先用丹宁酶处理,随后进行超过滤,最好再进行第二次过滤处理,最好用微过滤。
11 (1) There is also the possibility that the unusual chemical reactions that occur during irradiation may lead to the formation of chemicals that may be harmful to human. 然而,这种情况是可能的,即比起其它一些对健康有害的方法,辐射可更有效地杀死一些通常使得食物在变得不安全前就已变味的微生物。
(2) As far as is known vitamin C is not converted to substances which may prove harmful to man but the entire range of substances to which vitamins are converted has not yet to be identified. 正如已经知道的那样,维生素C 并不转化成对人体有害的物质, 然而,人们至今仍未弄清各种维生素的转化产物究竟有哪些。
(3) However it is possible that some micro-organisms which normally cause food to smell or taste bad before it has become unsafe may be destroyed more effectively by irradiation than those which are hazardous to health. 然而,很可能,与其它对健康有害的微生物相比,一些引起食物腐败变质的微生物在使食物变得不安全之前,更易有效地被辐射所杀灭。
(4) It is estimated that the lifetime dose of radioactivity to which a person eating irradiated food could be exposed could realistically amount to no more than one-millionth of the recognised limit of average exposure of an individual to radioactivity from other sources over the same period. 据估计,人们由于吃进辐射食物所遭受的放射剂量,实际上不多于同一时期一个人平均可能遭受到的来自其它放射源的、可觉察的放射的极限的百万份之一。
12 (1) The highly activated partially dissociative adsorption of nitrogen on ZSM-5 zeolite which is completely reversible at higher temperatures resembles to some extent the type C (M-N=N-M) nitrogen chemisorption on iron catalyst at lower temperatures. 氮气在ZSM-5型沸石上的高活化的部分解吸(这种解吸在高温下是完全可逆的)在某种程度上与C型氮( M-N=N-M ) 在低温时对铁催化剂的化学吸附相似。
13 (1) The use of lead dioxide in electrolytic systems, particularly for thermodynamic measurements, has indicated that irreproducible results are often obtained, and this imposes stringent purity requirements on the materials involved. 二氧化铅在电解体系,特别是需要进行热动力学测量的体系中的应用已经表明,所得的结果是有重现性的,这就对所涉及的材料的纯度提出了严格的要求。
14 (1) Nevertheless, Jorgensen`s contributions should not be slighted -- as an experimentalist he was second to none and had he not been prejudiced by some of the theories of valence current in his day, he might well have achieved the same results and fame as Werner. 然而,乔金森的贡献不能说不重要,他是唯一的第二个进行络合物实验研究的人,他若不是对一些在他那个时代盛行的价键理论太偏爱的话,他可能也会取得和沃纳一样的成果和名声。
(2) Werner, in formulating his ideas about the structure of coordination compounds, had before him facts such as the following. 沃纳在形成他的关于配位化合物结构的构想时,面对的是如下事实。
(3) From this conclusion, Werner postulated perhaps the most important part of his theory: that in this series of compounds cobalt exhibits a constant coordination number of 6 and that as ammonia molecules are removed, they are replaced by chloride ions which now act as though they are covalently bound to the cobalt, rather than as free chloride ions. 由此结论,沃纳提出了也许是他的理论中最重要的一部分:在该系列的化合物中,钴显示出固定为6的配位数,当消除了氨分子时,氯离子就取代了氨分子的位置并表现得仿佛是以共价键而不是以游离的形式和钴相结合。
15 (1) A battery comprises many ovens, each oven being heated indirectly by heat transferred through its refractory walls from flues on each long wall, in which coal gas is burnt under controlled conditions in air. 一套焦化设备是由许多高炉所组成的,其中每一个高炉的长壁上都安有烟道,煤气在这些烟道中在控制下在空气中燃烧,所产生的热通过其耐火壁对高炉进行间接加热。
(2) Air for combustion is drawn into the battery heating system, where it is heated by passing through flues in which gas was earlier burnt, then the preheated air is introduced into individual heating flues to support combustion of gas provided through jets. 燃烧所需的空气被引入焦化设备的加热系统,并使其通过其中已有燃烧着的煤气的烟道而被加热。然后,预热过的空气被导入各个加热烟道以维持由入口喷出的煤气的燃烧。
(3) A continuous purge is currently discharged to controlled waters and made up from water pumped from the site reservoir which is fed by mines drainage or from canal. 源源不断的水流被及时排泄到控制下的用水系统中,并由从现场的蓄水池中抽出的水加以补充,而蓄水池中的水则由矿区的排水系统或小渠供给。
16 The explosion of chemical information, the complexity of the information structure, the requirements to sophisticated instrumental facilities and the empirical nature of chemistry itself provide a wide stage of activities for computers, which are greatly enhanced by modern micro-electronics-based techniques in recent years. 化学情报的膨胀、情报结构的复杂性、对复杂仪器设备的要求以及化学本身的经验性都为计算机提供了广阔的活动舞台,而计算机近年来已被现代微电子技术大大加强了。
17 (1) You can be as selective as you need to be or as inclusive as you'd like to be. 你可以酯选取你所需的某些资料,也可以按你所喜欢的范围进行广泛的浏览。
(2) Science Citation Index, is one of the Institute for Scientific Information's multidisciplinary cited reference databases, provide efficient access to complete bibliographic data, full author abstracts, and cited references covering over 3,300 of the world's leading scientific and technical journals in a broad range of disciplines. 科学引文索引,是美国情报研究所多学科的引文数据库之一,它可有效地接近所有文献题录的数据、完整的作者摘要及3300多种多学科的、世界顶尖的科技杂志中所引用的文献参考。
18 The activity of catalyst particles sampled at different reactor positions after several reaction-regeneration cycles is related to their time-temp. history, according to the obtained sintering kinetics under coke-free conditions. 根据在无焦炭条件下所得的烧结动力学数据,在几次反应-再生循环之后,在反应器不同位置所取催化剂颗粒样品的活性与其使用的时间-温度情况有关。
19 Because MSA is non-complexing, it reduces the cost of complex waste treatment to bring tin and lead levels in the wastewater into compliance with environmental regulations. 因为MSA不会与金属离子形成螯合物,所以在进行复杂的后期废液处理,使废水中锡、铅含量达到环保标准时就可不用处理被螯合了的金属离子,这样就降低了复杂的后期废液的处理费用。
20 (1) The Buyer shall open, with a bank to be accepted by both the Buyer and the Seller, an irrevocable, transferable and divisible Letter of Credit at sight, allowing partial shipments and transhipment, in favour of the Seller (CHINA METALLURGICAL IMP. AND EXP. CORPORATION YUNNAN BRANCH) and negotiable against first presentation of the shipping documents to the Bank of China (YUNNAN BRANCH) in China. 买方应通过买卖双方同意的银行,开立以卖方(中国冶金进出口公司云南分公司 )为受益人的、不可撤销的、可转让和可分割的、允许分批装运和转船的即期信用证。 并可凭转运单据向中国银行云南省分行兑付现金。
(2) The Seller shall present to the negotiating bank, Clean On Board Bill of Lading (or Clean On Board Bill of Lading for container transportation), Quality certificate, Survey Report on Quantity/Weight, and Transferable Insurance Policy or Insurance Certificate when this Contract is made on CIF basis. 卖方应向兑付银行提供已装船提单( 或集装箱运输的已装船提单)、发票、品质证明、 数量/重量鉴定书。如果本合同按CIF结算, 应再提供可转让的保险单或保险凭证。
(3) The arbitration expenses shall be borne by the Losing party unless otherwise awarded by the arbitration organization. 仲裁决定是终结的,对双方具有同等约束力。仲裁费用除非仲裁机构另有决定外,均由败诉一方负担。
编辑推荐:
下载Word文档
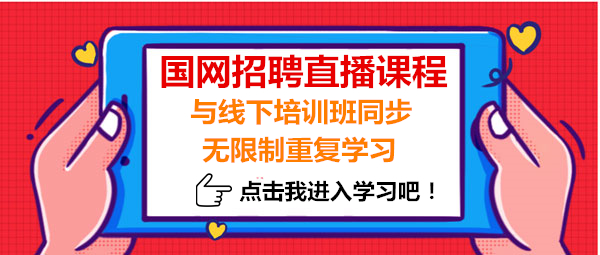
温馨提示:因考试政策、内容不断变化与调整,长理培训网站提供的以上信息仅供参考,如有异议,请考生以权威部门公布的内容为准! (责任编辑:长理培训)
点击加载更多评论>>